Advances in the Global Distribution, Chemical Constituents, and Pharmacology of Hippocampus, a Traditional Marine Chinese Medicine
- 1College of Pharmacy, Shandong University of Traditional Chinese Medicine, Jinan, China
- 2Marine Traditional Chinese Medicine Research Center, Qingdao Academy of Traditional Chinese Medicine, Shandong University of Traditional Chinese Medicine, Qingdao, China
- 3Institute of Traditional Chinese Medicine Literature and Culture, Shandong University of Traditional Chinese Medicine, Jinan, China
- 4Shandong Engineering and Technology Research Center of Traditional Chinese Medicine, Jinan, China
Hippocampus is an important traditional marine Chinese medicinal resource that has been used to warm and tonify kidney yang in the clinic for a long time in China. Modern pharmacological studies show that its active ingredients display a wide range of pharmacological activities associated with the kidney, such as anti-inflammation, antioxidation, antitumor, and neuroprotective effects. Herein, we systematically summarize and analyze the research progress on the resource distribution, active ingredients, pharmacological activities, and clinical application of Hippocampus. First, the species and worldwide distribution of Hippocampus were assessed to clarify the existing resources, and the results showed that 44 species of Hippocampus have been found in 159 countries and regions worldwide. Then, based on the analysis of 16 kinds of active ingredients and extraction methods, the relationship between the ingredient extraction and pharmacological activities of Hippocampus was revealed. This review may provide a foundation for further research on the potential active ingredients and mechanisms of Hippocampus. In addition, the research status of traditional prescriptions containing Hippocampus was evaluated. The results implied that research on Hippocampus is still in its infancy, and the mechanism and material basis of its efficacy have not been clarified. This paper should provide directions for further studies on Hippocampus.
Introduction
The ocean is rich in biodiversity, and many organisms have evolved unique morphological and physiological characteristics to adapt to challenging environments (Conte et al., 2021). Marine organisms further produce marine natural products (MNPs) with chemical diversity and interesting structures, including peptides, terpenoids, steroids, polyphenols, alkaloids, etc., (Sable et al., 2017; Murray et al., 2018; Wu et al., 2018; Carroll et al., 2019; Althagbi et al., 2020; Ermolenko et al., 2020). Currently, thousands of new MNPs are being reported every year (Carroll et al., 2020, 2021). These compounds appeared to be promising therapeutics for the treatment of a variety of human diseases, such as cancers and cardiocerebrovascular diseases (Dayanidhi et al., 2021; Liang et al., 2021). In addition, studies have shown that the molecular weight and clog P of MNPs isolated since 1957 were particularly similar to that of approved drugs (Blunt et al., 2018). MNPs have always been an important source of new medicines (Khalifa et al., 2019). Currently, there are seven FDA-approved medicines and more than 20 medicine candidates from 25,000 NMPS; thus, MNPs have a bright future compared with synthetic molecules (Newman and Cragg, 2016). Marine biodiversity may afford potential resources for new NMPS.
Organisms of the genus Hippocampus (Syngnathidae) are widely distributed in marine ecological systems. As a traditional Chinese medicine (TCM), Hippocampus was first recorded in a Supplement to Materia Medica (Bencao Shiyi), which has been clinically applied for thousands of years (Chen, 1983). There are five medicinal Hippocampus organisms recorded in the Chinese Pharmacopoeia: Hippocampus kuda Bleeker, Hippocampus trimaculatus Leach, Hippocampus kelloggi Jordan et Snyder, Hippocampus histrix Kaup, and Hippocampus japonicus Kaup (Chinese, 2020). In TCM, the main efficacy of Hippocampus is warming and tonifying of the kidney yang. Modern pharmacological studies have reported that Hippocampus shows various biological activities, including antifatigue, anti-inflammation, antioxidation, antitumor, and antimicrobial activities (Kumaravel et al., 2012). Peptides, steroids, and fatty acids are the main active ingredients of Hippocampus. In addition, nucleosides, phthalates, ketones, etc., have also been isolated and identified (Chen et al., 2015). These results supported the great therapeutic potential of Hippocampus.
In this study, the research progress on the resource distribution, active ingredients, pharmacological activities, and prescriptions of Hippocampus was systematically analyzed and summarized. According to the literature, websites, and databases, we reviewed 44 Hippocampus species and their distributions worldwide, and resources for medicinal Hippocampus were emphasized. Sixteen kinds of ingredients of Hippocampus were described, and possible Hippocampus factors were investigated, such as the species, growth stage, wild or culture, and bait. In addition to traditional efficacy, more attention has been given to modern pharmacological agents, especially neuroprotective agents. Moreover, studies on the relationship among ingredients, extraction methods, and pharmacological activities may help to identify new active ingredients to supplement the compound-constituent groups of Hippocampus, which may further provide a scientific basis for explaining its mechanism in treating diseases. In addition, the clinical application of traditional prescriptions containing Hippocampus also may provide new ideas for the development and utilization of Hippocampus in the future.
Status of Hippocampus Resources
Hippocampus Distribution and Species Worldwide
At present, 44 species of Hippocampus have been found in 159 countries and regions worldwide (Figure 1 and Supplementary Table 1; CITES, 2021b; FishBase, 2021), with the highest diversity of species observed in Australia (Figure 2A). Oceania has the largest number of species on the continent, followed by Asia and Africa (Figure 2B). Hippocampus is primarily found in the Indo-Pacific Ocean and distributed in shallow waters with a depth of less than 30 m in temperate, subtropical, and tropical regions (Foster and Vincent, 2004). On the west coast of peninsular Malaysia, Hippocampus kelloggi Jordan et Snyder was collected by trawlers in deepwater below 65 m (Choo and Liew, 2003). The habitat of Hippocampus includes seagrass, coral reefs, mangroves, and seaweeds, with coral reefs representing the main habitat constituents of tropical Hippocampus (Foster and Vincent, 2004). Hippocampus coronatus Temminck and Schlegel and Hippocampus mohnikei Bleeker generally inhabit seagrass in the southern coastal waters of Korea. Temperate Hippocampus tends to inhabit seagrass beds, which provide food for a variety of flora and fauna (Choi et al., 2012). Among the five medicinal Hippocampus in China, only Hippocampus japonicus Kaup is mainly distributed in northern China. The other four species were mainly found in the tropical and subtropical regions of China, such as the East China Sea and South China Sea (Lin et al., 2008; Han, 2013).
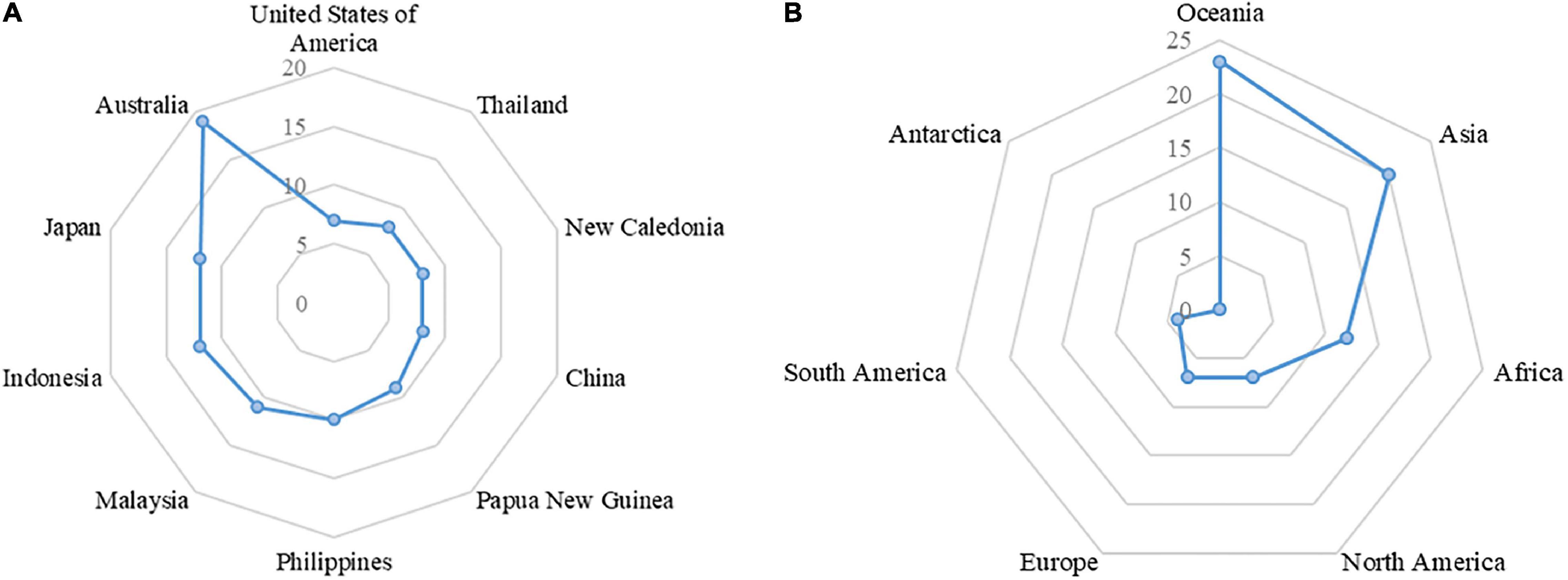
Figure 2. (A) The top 10 countries of Hippocampus species; (B) The species of Hippocampus in each continent.
The ease of identification of a Hippocampus specimen varies from species to species. Hippocampus specimens were generally identified using preparation tools (ruler, magnifying glass, calculator, etc.) by identifying characteristics, using measured and recorded data about an unidentified specimen, and using standards (Supplementary Tables 1–6 from a guide of Hippocampus identification) to determine possible species and identify specimens. The data mainly included the head length (HL) and snout length (SnL), length of the snout in relation to the length of the head (HL/SnL), number of tail rings (TaR), trunk rings (TrR), etc., (Lourie et al., 2004). The characteristics and morphological identification based on distinguishing characteristics are appropriate when performed by investigators who are experienced with Hippocampus identification. However, some Hippocampus specimens are particularly similar, and new species may be encountered; thus, deoxyribonucleic acid (DNA) barcoding technology may need to be applied for Hippocampus identification. The species phylogenetic tree is constructed based on the alignment results of the complete mitochondrial genome or mitochondrial cytochrome b gene from different species (Casey et al., 2004; Ge et al., 2018). The phylogenetic tree with the Cytochrome Oxidase I (COI) gene as a primer showed that different species of Hippocampus were in different branches with reasonable distances between them, and the accuracy was higher than that of other mitochondrial genes (Hou et al., 2016). Liu et al. (2018) used a multiplex polymerase chain reaction (PCR) method to simultaneously identify five medicinal Hippocampus species by judging the absence and size of DNA bands on the gel map in a single assay. DNA barcoding provides an accurate technique for the identification of Hippocampus specimens. A rapid detection kit should be developed in the future to reduce the complicated and time-consuming aspects of this technology. Fast and effective identification methods can be used to protect endangered Hippocampus species and monitor the Hippocampus trade (Luo et al., 2013). In TCM, metabolomics technology has been used to study differences in metabolites for various species and find metabolic markers, and such work has provided references for the identification of multisource medicinal materials (Fan, 2012). This may provide new ideas for the identification of Hippocampus.
Global Trade Volume of Hippocampus
Recent studies have revealed that the number of Hippocampus individuals has continuously declined over the past 20 years. The trade demand for Hippocampus has increased significantly because of its value for medicine, aquarium displays, and collectibles (Vincent et al., 2011). The export volume of medicinal Hippocampus is concentrated in Southeast Asian countries, with Thailand being the largest exporter (Figure 3A and Supplementary Table 2; CITES, 2021a). The export volume of Hippocampus kuda Bleeker, Hippocampus trimaculatus Leach, and Hippocampus kelloggi Jordan et Snyder accounted for 88% of the global export of dried Hippocampus (Loh et al., 2016). Regarding importing countries, China and its affiliated Hong Kong Special Administrative Region and Taiwan were the main importers of dried Hippocampus due to the influence of TCM (Figure 3B and Supplementary Table 3; CITES, 2021a). Exports of other Hippocampus species were mainly concentrated in Australia and some countries in the Americas (Convention on International Trade in Endangered Species of Wild Fauna and Flora, 2020). A large global trade volume is mainly associated with wild Hippocampus caught by trawl by catch. Huge fishing pressure has made Hippocampus populations unsustainable (Vincent et al., 2011). Moreover, the Hippocampus population is also threatened by habitat reduction and environmental pollution (Rosa et al., 2005). The International Union for Conservation of Nature (IUCN) listed Hippocampus on the red list, with five medicinal Hippocampus species listed as vulnerable species (IUCN, 2021). In addition, breeding techniques for Hippocampus similar to wild medicinal species are required. With the gradual solution to problems associated with rearing and bacterial infection in the breed, an increasing number of countries have tried to breed Hippocampus, such as Australia, Brazil, China, Sri Lanka, the United Kingdom, the United States, and Vietnam (Koldewey and Martin-Smith, 2010). The amount of wild Hippocampus cannot meet market needs, and artificial breeding is an important way to meet market demand and protect wild Hippocampus resources. In addition, we may seek alternative species. The phylogenetic tree of Hippocampus listed eight species of Hippocampus that were similar to the medicinal Hippocampus (Figure 4 and Supplementary Table 4; NCBI, 2020). As a next step, we may identify Hippocampus species with a large trade volume to study their ingredients and pharmacological activities and strive to find supplemental medical Hippocampus species.
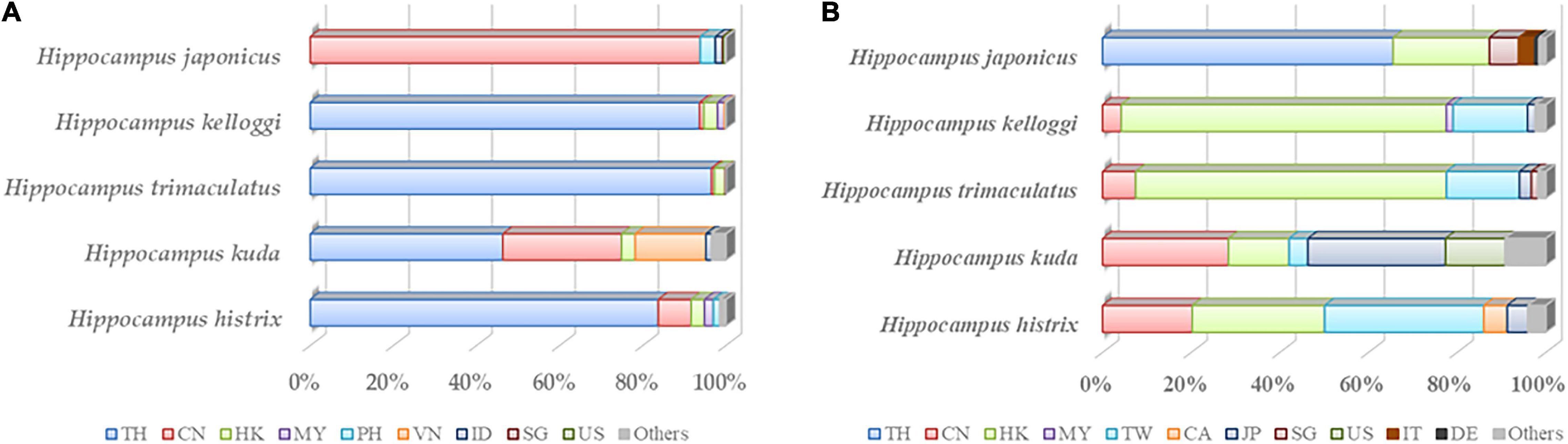
Figure 3. (A) In five medicinal Hippocampus, the export volume of the top five countries and regions accounted for proportion of total export volume in 2000–2020. (B) In five medicinal Hippocampus, the import volume of the top five countries and regions accounted for proportion of total import volume in 2000–2020. TH, Thailand; CN, China; HK, Hong Kong (China); MY, Malaysia; PH, Philippines; VN, Vietnam; ID, Indonesia; SG, Singapore; US, United States of America; TW, Taiwan Province of China; CA, Canada; JP, Japan; IT, Italy; DE, Germany; Others, other countries and regions.
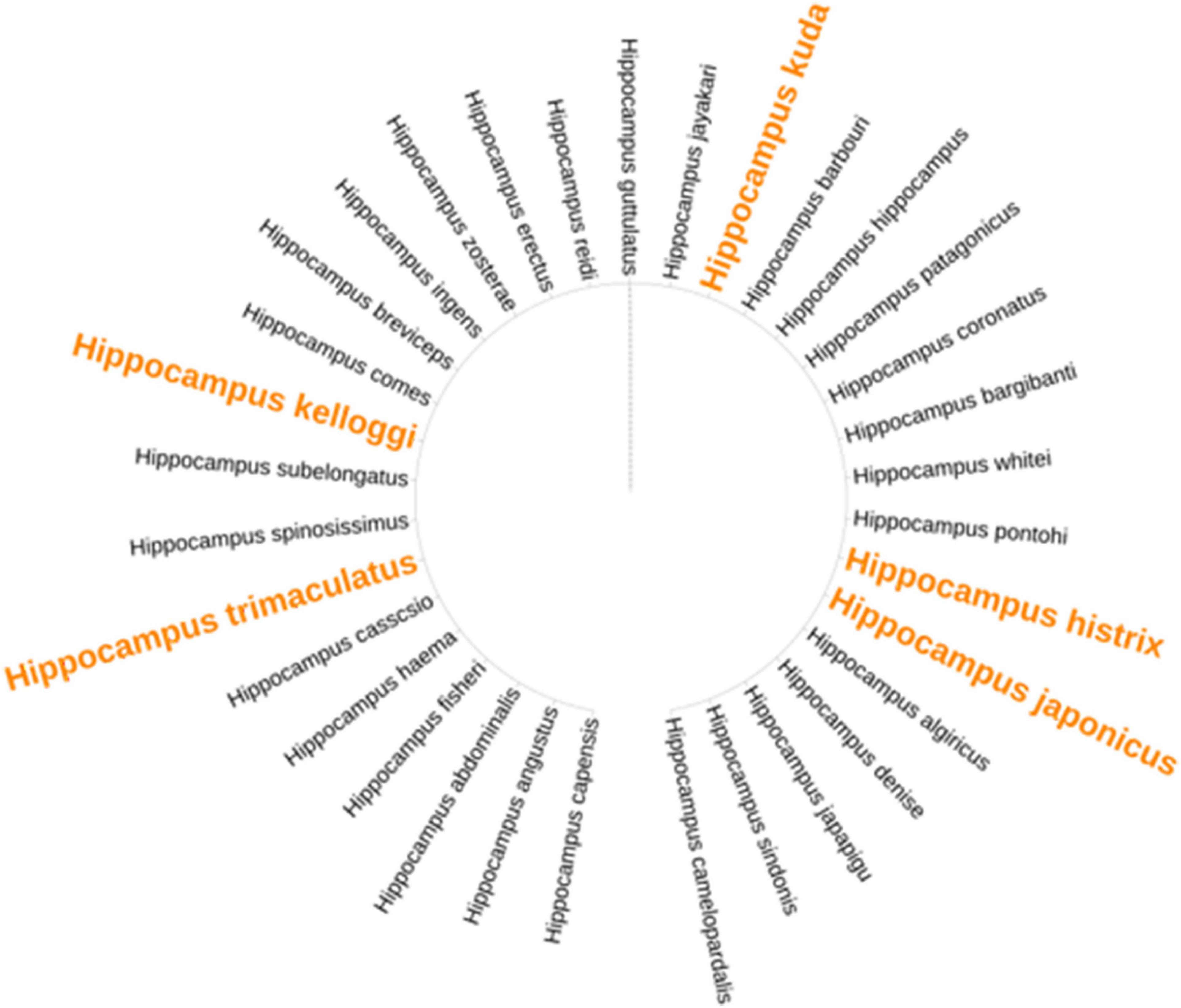
Figure 4. The phylogenetic tree of 33 species of Hippocampus. Yellow fonts represent the medicinal Hippocampus.
Traditional Medicinal Use
The traditional usage of Hippocampus was recorded in the Chinese Materia Medica (Zhonghua Bencao), and it indicates that Hippocampus was extracted with water to make a decoction, in which the dosage of Hippocampus was 3–9 g. The decoction was taken for its ability to warm and tonify kidney yang and reduce swelling. In addition, Hippocampus was further processed into a powder for external use to treat furunculosis (Song, 1999). The Chinese Pharmacopoeia also included this traditional extraction method and dosage (Chinese, 2020). At present, relevant reports have not indicated that Hippocampus is harmful to the human body. However, few studies have performed safety assessments of Hippocampus (Zhu, 2004).
In the Ming dynasty, “warming kidney, tonifying yang, eliminating lumps, and curing carbuncle” were the efficacies of Hippocampus recorded in The Compendium of Materia Medica (Bencao Gangmu; Li, 1982). The traditional efficacy of warming and tonifying kidney yang is not only reflected in its ability to improve reproductive function but also in its ability to regulate organs related to the kidney (Zhao et al., 2021). Kidney and yang have unique meanings in terms of TCM theory. The kidney may be understood from two aspects. First, in addition to referring to the organ in Western medicine, the kidney is also associated with a series of internal organs, namely, the kidney, bladder, bone, brain, ear, etc. Second, e kidney has effects on reproduction and operates in conjunction with other organs to complete the metabolism of the body (Wang et al., 2012). Yin and yang theory has been used to explain diseases that occur in the body (Ji and Yu, 2020). Yang has effects of warming, exciting, pushing, dispersing, and lifting in the human body (Zhang, 2021). Therefore, kidney yang deficiency causes the human body to have symptoms that include sperm deficiency, soreness and weakness of the waist and knees, dizziness, and tinnitus. These symptoms correspond to kidney disease and reproductive, immune, nervous, and other diseases in modern medicine (Qi et al., 2012; Reheman et al., 2019; Tian et al., 2021). In modern studies, the pharmacological activities of Hippocampus, such as its antihypertensive, antifatigue, and neuroprotection activities, have been reported and are consistent with traditional theory (Chen et al., 2015).
Traditional prescriptions containing Hippocampus were commonly made into pills, capsules, plasters, and wines for medicinal usage. The main clinical efficacies of the pill and capsule forms were warming and tonifying kidney yang, such as Haimabushen pill, Haimaduobian pill, and Hippocampus capsule (Supplementary Table 5). In TCM, pills have delayed release in the gastrointestinal tract to achieve stable and long-lasting efficacy and reduce toxicity and adverse reactions (Zhang et al., 2017). Pills containing Hippocampus might be suitable for chronic diseases of kidney deficiency. Haimabushen pill enriches Yin and tonifies the kidney and improves fatigue, soreness, and weakness in the waist and knees. Zhi et al. (2019a) found that Haimabushen pill improved the testosterone content level and had androgen-like effects in castrated or young mice by regulating the hypothalamus-pituitary-gonad axis. Additionally, Haima Zhuangyang soft capsule significantly shortened the incubation period for the erection of the penis and increased the prostate and semen sac coefficients of kidney yang-deficient mice (Lu et al., 2001). Capsules with controllable quality have been made by modern advanced preparation technology. Compared with traditional pills, the reproductive organ index of ovariectomized mice treated with low-dose Haima capsules (0.045 g/kg) was significantly higher than that of ovariectomized mice treated with middle-dose Haimabushen pill (0.28 g/kg) (Mei et al., 2005). In addition to the traditional effect of warming and tonifying kidney yang, Hippocampus in the plaster form was often compatible with medicines for dispelling wind and drying dampness. In particular, plasters are preferred formulations to treat local symptoms and quickly relieve the pain to improve the treatment comfort of the patient (Jia et al., 2003). For example, Shexiang Haima Zhuifeng Gao has been used to treat wind and dampness impediments and lumbago and leg pain (Liu and Lin, 2006). Medicinal wine may exert the biological effects identified in the trace elements in Hippocampus better than a decoction. Research has shown that seahorse medicinal liquor contains amino acids and mineral elements, has antifatigue effects, and improves immunity (Huang et al., 1997).
Traditional prescriptions containing Hippocampus are widely used in the clinic, and their pharmacological effects have gradually attracted more attention. Traditional prescriptions containing Hippocampus have been further explored using animal experiments to identify other effects, such as in learning, memory, and tumors. In a mouse model of memory impairment induced by scopolamine and sodium nitrite, Haimabushen pill effectively reduced the impairment of learning and memory ability of mice through its acetylcholinesterase inhibitory ability (Zhi et al., 2019b). Haimashengshui pill was also found to inhibit S180 tumor-bearing mice and promote tumor cell apoptosis (Han, 2002). The formulation of traditional prescriptions containing Hippocampus has become an urgent issue. New formulations are another main method of expanding the clinical application of traditional prescriptions containing Hippocampus. For example, nanotechnology has the ability to cross various biological barriers (De Jong and Borm, 2008). The nanoparticle formulation of Haimabushen pill may play a greater role in crossing the blood–brain barrier and entering the brain. In addition, the Hippocampus species used in traditional prescriptions are not fully understood, which may have a certain impact on clinical efficacy.
Active Ingredients of Hippocampus
Many Hippocampus species in China have been chemically investigated. Peptides were identified from Hippocampus located in Indonesia, South Korea, and China. Steroids were mainly obtained from species in China and Australia. Although the main source of fatty acids is China, these ingredients were also found in Mexico and Spain (Figure 5). To date, a total of 329 ingredients in 16 categories have been identified, including acids, steroids, amino acids, mineral elements, nucleosides, phospholipids, phthalates, peptides, glycoproteins, volatile ingredients (hydrocarbons, alcohols, aldehydes, ketones, esters, amines), and others (Supplementary Figure 1 and Supplementary Table 6).
Amino Acids and Peptides
Amino acids play vital roles in regulating metabolism, growth, and development. Among the 20 types of amino acids constituting human proteins, essential amino acids cannot be synthesized by humans (Mitsuhashi, 2014). Twenty-three types of amino acids have been found in Hippocampus (Supplementary Table 6). Lin et al. (2008) found that the total amount of amino acids was as high as 50–60% (based on a comparison of their retention times with those of amino acid standards) in six species of Hippocampus, and eight types of essential amino acids accounted for 17–20% of the total amino acid content, which indicated that the Hippocampus is a high-quality protein source. The content of glycine was the highest (83.98 mg/g) among the 15 types of amino acids in Hippocampus kuda Bleeker and Hippocampus trimaculatus Leach (Sun et al., 2020). In addition, Hippocampus is also rich in glutamic acid, aspartic acid, alanine, and arginine (Lin et al., 2009). Jia and Yao (1990) reported 22 types of amino acids in Hippocampus kuda Bleeker and Hippocampus japonicus Kaup, including taurine, a special amino acid with high medicinal value. The type and the content of amino acids in Hippocampus erectus Perry were similar to those in medicinal Hippocampus, and even the content of essential amino acids in Hippocampus erectus Perry was higher than that in medicinal Hippocampus (Yan Z. Z., 2019). Peptides are important marine natural products that widely exist in vast marine organisms. Most peptides extracted from Hippocampus have various pharmacological activities and can be classified as angiotensin-converting enzyme (ACE) inhibitory peptides, anti-inflammatory peptides, neuroprotective peptides, and antimicrobial peptides. Shi et al. (2020) isolated ACE inhibitory peptides with the amino acid sequence Pro-Ala-Gly-Pro-Arg-Gly-Pro-Ala from the enzymatic hydrolysate of Hippocampus trimaculatus Leach. Circular dichroism analysis showed that the secondary structure was mainly composed of a random coil. Three ACE inhibitory peptides were also isolated from cultured Hippocampus abdominalis Lesson. The amino acid sequence of Cys-ASN-Val-Pro-Leu-Ser-Pro was more stable in molecular docking with ACE, which may become the preferred candidate for antihypertensive peptides (Je et al., 2020). Ryu et al. (2010) isolated an anti-inflammatory peptide composed of 15 types of amino acids (including five aspartic acids) from Hippocampus. Recently, a novel neuroprotective peptide (HTP-1) rich in hydrophobic amino acids and a molecular weight of less than 1 kDa have been isolated from Hippocampus trimaculatus Leach. The N-terminal amino acid sequence of HTP-1 is similar to that of insulin-like growth factor-1 (IGF-1) and may have neuroprotective effects (Pangestuti et al., 2013). Sun et al. (2012) identified an antimicrobial peptide (HKPLP) from the brooding pouch of male Hippocampus Kuda Bleeker. Bioinformatics analyses of available genomic and transcriptomic data allowed us to find the distribution of antimicrobial peptides closely related to the male brooding pouch (Chen et al., 2019). In conclusion, the activity of low molecular weight peptides is affected by the composition and sequence of amino acids. In terms of favorable structure-activity relationships for high ACE-inhibitory activity, aromatic amino acids at the C-terminus end and hydrophobic amino acids at the N-terminus end were most potent (Xu, 2015). 2,2-Diphenyl-1-picrylhydrazyl (DPPH) radical scavenging results indicated that low molecular weight (43–278 kDa) peptides from Hippocampus erectus Perry showed higher activity than peptides over 278 kDa (Chen et al., 2018). Elucidating the structure-activity relationships of these peptides may help develop peptides with enhanced bioactivity.
Steroids
Steroids are among the major ingredients of Hippocampus, and 38 types of steroids have been isolated and identified to date (Supplementary Table 6). Gas chromatography–mass spectrometry (GC–MS) analysis identified 16 types of steroids from seven species of Hippocampus. Cholesterol and cholest-4-en-3-one were the major ingredients in the total steroids (Chen, 2015). Sun et al. (2020) reported that the average content of steroids in Hippocampus kuda Bleeker was 6.16 mg/g, and cholesterol accounted for 0.55 mg/g. The average ratios of cholest-4-en-3-one and cholest-3,6-dione in Hippocampus trimaculatus Leach, Hippocampus histrix Kaup, and Hippocampus japonicus Kaup were 0.0301 and 0.0096%, respectively (Zhang et al., 1998). In addition, Wu et al. (2017b) extracted 3β-hydroxycholest-5-en-7-one and cholest-5-en-3β,7β(7α)-diol from Hippocampus trimaculatus Leach. Cholesterol stearate and cholest-5-en-3β,7α-diol were also extracted from Hippocampus histrix Kaup (Wang et al., 1998). Yang et al. (2014) supplemented steroids of Hippocampus erinaceus Gunther, and six ingredients were identified: cholesterol, cholest-4-en-3-one, (22E,24R)-3β,5α,9α-trihydroxyergosta-7,22-dien-6-one, 24-methyl-cholesta-7,22-diene-3β,5α,6β-triol, 3β-hydroxy-7-methoxy-cholesta-5-en, and 3β-hydroxycholest-5-en-7-one. In addition, brassicasterol, which has antitumor activity, has been recently obtained from Hippocampus abdominalis Lesson (Xu et al., 2020). Although a large number of steroids have been isolated, marker ingredients have not been identified from Hippocampus quality evaluation standards. It is urgent to establish a stable and controllable method to scientifically evaluate and control the quality of Hippocampus.
Fatty Acids
Hippocampus contains 82 types of fatty acids, including 35 types of saturated fatty acids and 47 types of unsaturated fatty acids (Supplementary Table 6). Lin et al. (2008) found that there were 12 types of fatty acids distributed widely among six species of Hippocampus, and hexadecanoic acid, octadecanoic acid, oleic acid, 5,8,11,14,17-eicosapentaenoic acid (EPA), and 4,7,10,13,16,19-docosahexaenoic acid (DHA) were the main ingredients. The ratios of unsaturated fatty acids and EPA and DHA to total fatty acids were 48–59% and 14–35%, respectively. Studies have shown that EPA and DHA not only reduce the risk of cardiovascular diseases, such as atherosclerosis, arrhythmia, and hyperlipidemia (Watanabe and Tatsuno, 2017), but also improve sperm quality and sperm motility when used as food supplements (Hosseini et al., 2019). Chen (2015) identified 26 types of fatty acids from seven species of Hippocampus; among benzenepropanoic acids, 3,5-bis(1,1-dimethylethyl)-4-hydroxy was found for the first time. Tetradecanoic acid, hexadecanoic acid, octadecanoic acid, 9(Z)-octadecenoic acid, 5,8,11,14-eicosatetraenoic acid, and DHA were the main fatty acids in the five medicinal Hippocampus species. Zhang and Wang (1996) isolated 42 fatty acid peaks of Hippocampus japonicus Kaup by a rapid fat extraction method, among which EPA and DHA accounted for 14.1%. Amazingly, there were many other types of fatty acids in Hippocampus erectus Perry, such as heptadecanoic acid, tetracosenoic acid, 11-octadecenoic acid, 15-tetracosenic acid, and 7,10,13,16-docosatetraenoic acid (Nicolás et al., 2014).
Mineral Elements
Mineral elements play an important role in growth because they help to improve immunity and anti-fatigue (Sherman, 1992). Twenty-four types of mineral elements were found in five Hippocampus medicinal species. The contents of P, K, Na, and Mg were all above 100 mg/g, while the contents of mineral elements, including Al, Fe, Mn, Sr, Zn, etc., were at the level of a microgram. Lin et al. (2009) reported that the contents of Mn and Zn in Hippocampus trimaculatus Leach were 21.73 and 31.44 μg/g, respectively. The total contents of mineral elements in Hippocampus Kuda Bleeker were 84,873 μg/g, which also contained Cr, Co, Se, and Pb (Jia and Yao, 1990). The effect of Hippocampus on warming and tonifying kidney yang also benefited from mineral elements (Zhang et al., 1997). Clinical studies have shown that higher levels of mineral elements may reduce the likelihood of declines in cognitive function over 5 years (Gerardo et al., 2020). In the brain, various nervous activities require the participation of essential mineral elements, such as Fe and Zn. Some neurodegenerative diseases are related to an imbalance in the homeostasis of these cations (Bohic et al., 2011), which indicates that Hippocampus may have a therapeutic effect on neurodegenerative diseases.
Phospholipids and Nucleosides
Studies on Hippocampus mainly focus on amino acids, mineral elements, and fatty acids, while relatively few reports have focused on the analysis technologies and ingredient activities, such as that of phospholipids and nucleosides. Phospholipids are the main component of biomembranes. A study of the contents and types of phospholipids in five medicinal Hippocampus was carried out using molybdenum blue colorimetry and thin layer chromatography (TLC) scanning. The results showed that the total contents ranged from 3.28 to 7.82 mg/g, including LPC, SM, PC, and PE (Xu et al., 1994). Shen et al. (2016) identified 50 phospholipid molecules by hydrophilic interaction chromatography-quadrupole time of flight-mass spectrometry (HILIC-QTOF/MS). In addition to PC and PE, there were 12 PIs and 9 PSs. PC and PE accounted for most of the total phospholipids at 1.91–4.89 mg/g and 1.52–2.64 mg/g, respectively. Marine nucleosides were found to have antiviral, anticancer, and hypertensive activities (Huang et al., 2014). Zheng et al. (2012) reported that the contents of hypoxanthine extracted from Hippocampus Kuda Bleeker and Hippocampus japonicus Kaup were 0.73 and 0.52 mg/g, respectively. The average contents of nucleosides and nucleotides in the Hippocampus japonicus Kaup were 1.974 and 0.79 mg/g, respectively. However, uridine, cytosine nucleotides, and guanine nucleotides were not found in Hippocampus japonicus Kaup (Wei et al., 2015). Yan Z. Z., (2019) found six nucleosides in Hippocampus erectus Perry, more types and contents than those in Hippocampus japonicus Kaup.
Other Ingredients
Apart from the abovementioned ingredients, other ingredients have also been reported. Six phthalate derivatives have been isolated from ethanol extracts of Hippocampus Kuda Bleeker and Hippocampus trimaculatus Leach, including dibutyl phthalate, bis(2-ethylhexyl)phthalate (Wu et al., 2017b), bis(2-ethylheptyl)phthalate, bis(2-ethyldodecyl) phthalate, 2-ethyldecyl 2-ethylundecyl phthalate, and 2,12-diethyl-11-methylhexadecyl 2-ethyl-11-methylhexadecylphthalate (Li et al., 2008). As a part of ongoing research on extraction and isolation, paeonol was incidentally isolated from Hippocampus Kuda Bleeker (Himaya et al., 2012). The flavor profile was identified based on a set of volatile ingredients that rendered the characteristic taste and smell of Hippocampus. Based on headspace solid-phase microextraction combined with GC–MS, 84 potential volatile ingredients were identified, including acids, hydrocarbons, alcohols, aldehydes, ketones, esters, and amines. The main flavor ingredients with contributions over 70% were (E,E)-3,5-octadiene-2-one, trimethylamine, hexanoic acid, 2-nonone, etc., (Si et al., 2018). Glycoproteins of Hippocampus have rarely been reported because of their relatively complex composition. Box-Behnken and ultrasonic extraction technology were applied to separate glycoproteins HG-11 and HG-21 (Su and Xu, 2015a). Glycoproteins included in marine organisms are the main active ingredients and deserve additional attention in future research. In addition, the nuclear magnetic resonance (NMR) and GC–MS metabonomics methods (Zhao, 2018) and the Traditional Chinese Medicines Integrated Database (TCMID) (TCMID, 2020) have provided a large amount of information regarding the chemical ingredients of Hippocampus.
Influencing Factors of Different Ingredient Constituents
The ingredient constituents of Hippocampus are affected by multiple factors. Different species of Hippocampus have significant differences in ingredient constituents (Lin et al., 2008). Studies on the influence of sex and wild and cultured growth stages on Hippocampus ingredients provided a basis for medicinal research and breeding technology (Su and Xu, 2015b). Based on the connection of factors and constituents, Hippocampus species may be chosen according to the needs of the research. We may adjust the proportion of baits in breeding according to the difference in ingredient constituents. In contrast, such studies have also provided references for species identification. The ingredient constituents in the samples may also provide information on the Hippocampus species, genders, growth stage, etc.
Species and Genders
Lin et al. (2008) compared different ingredient constituents of six species of Hippocampus. The crude protein content was the most abundant in Hippocampus kelloggi Jordan et Snyder, while, in Hippocampus histrix Kaup, was the lowest. Notably, more than half of the fatty acids in Hippocampus comes Cantor were unsaturated fatty acids, resulting in approximately 1.25–1.8 times as much EPA and DHA in Hippocampus comes Cantor compared to those in medicinal Hippocampus species. Mg was the most abundant mineral element in Hippocampus trimaculatus Leach and Hippocampus histrix Kaup, and the contents were 1125.44 and 1076.57 μg/g, respectively. Chen (2015) compared steroids in seven species of Hippocampus. The most abundant steroids content was found in Hippocampus japonicus Kaup, and the least abundant steroid content was found in Hippocampus histrix Kaup and Hippocampus comes Canter. Xu et al. (1994) reported the total phospholipid contents of five medicinal Hippocampus species. The highest phospholipids content was observed in Hippocampus japonicus Kaup (7.82 mg/g), but PEs were hardly detected compared with that of any other Hippocampus species. 1H-NMR metabonomics studies showed that the contents of glutamic acid, aspartic acid, glycerol, taurine, and acetic acid in Hippocampus trimaculatus Leach and Hippocampus kelloggi Jordan et Snyder were significantly higher than those in Hippocampus kuda Bleeker, while the contents of isoleucine and leucine were significantly low (Zhao, 2018). TCM theory about medicinal Hippocampus emphasizes that both male and female Hippocampus should be used together (Xu et al., 2002). Whether there was a difference in medicinal value between males and females was not clear. At the age of 6 months, female Hippocampus had a slightly higher proportion of steroids and unsaturated fatty acids than males (Sun et al., 2020). The fatty acid extraction rate of male Hippocampus was slightly higher than that of females (Su and Xu, 2015b). There was no significant difference in ingredient constituents between male and female Hippocampus erectus Perry and Hippocampus trimaculatus Leach, although the contents of cholesterol and hypoxanthine in female Hippocampus were significantly higher than that in males, and the content of uridine in female Hippocampus erectus Perry was significantly lower than that in males (Yan Z. Z., 2019). Such findings may explain why male and female Hippocampus would be used together. In conclusion, gender had a limited effect on the ingredient constitutions.
Growth Stage
Eggs of Hippocampus guttulatus Cuvier are round and transparent, and the yolk sac provides nutrition for embryo development. Eggs and newborn Hippocampus represent different growth stages, and their ingredient constituents are different. Taking fatty acids as an example, the fatty acid contents in newborn Hippocampus were much lower than those in eggs. The content of unsaturated fatty acids in eggs was more than four times higher than that in newborn Hippocampus, and DHA and EPA were rich in eggs. Omega-3 unsaturated fatty acids were consumed during embryonic development, which explained the difference in fatty acid contents between newborn Hippocampus and eggs (Faleiro and Narciso, 2010). Sun et al. (2020) analyzed the ingredients of wild Hippocampus at the ages of 6 month, 1 year, and 2 years. It was obvious that crude protein content was higher in the ration of 1-year-old Hippocampus compared with the ration of 6- and 2-year-old. The Fe, Cu, and Zn contents increased with age, whereas unsaturated fatty acid contents decreased significantly. Contents of proteins and fatty acids were more abundant in 1-year-old Hippocampus; therefore, Hippocampus in this stage is proper for TCM applications.
Wild Types and Cultured Types
The qualities of wild and cultured Hippocampus differed due to the different growth environments. The contents of essential amino acids and unsaturated fatty acids were much higher in cultured Hippocampus kuda Bleeker than in wild Hippocampus. In terms of mineral elements, Se, Fe, Cu, and Ca were much more abundant in wild Hippocampus (Sun et al., 2020). Lin et al. (2009) showed that the contents of DHA and EPA in cultured Hippocampus trimaculatus Leach were nearly two times as high as those in wild Hippocampus, and the proportions of polyunsaturated fatty acids in total fatty acids were 31.89 and 21.39%, respectively. The cultured Hippocampus was rich in polyunsaturated fatty acids, but there were some deficiencies in protein. In addition to Pb, the contents of Cd, Cr, Zn, and Mn in cultured Hippocampus were higher than those in wild Hippocampus. Additional nutritional supplements were added to the bait as required. Understanding the difference in nutritional characteristics between wild and cultured Hippocampus is helpful for optimizing the breeding technology and supplementing wild Hippocampus.
Baits
For cultured Hippocampus, different baits had a great influence on the ingredient constituents of Hippocampus. Hexadecanoic acid, octadecenoic acid, octadecatrienoic acid, eicosapentaenoic acid, etc., are rich in Artemia and rotifers. Several differences in fatty acid types have been observed for different baits, and obvious variations have been observed for EPA and DHA. Otero-Ferrer et al. (2010) fed Hippocampus hippocampus Linnaeus Artemia and rotifers, and, after 5 days of feeding, the rotifer group showed a higher level of omega-3 unsaturated fatty acids, and the fatty acid types were more abundant than those in the Artemia group. The contents of 9,12-octadecadienoic acid and 9,12,15-octadecatrienoic acid in the Artemia group were more prominent. After 34 days of feeding, fatty acids in the Artemia group were gradually enriched, and the contents of DHA and EPA in the Artemia group were higher than those in the rotifer group. In addition, the Artemia group also showed lower mortality and a higher growth rate. Segade et al. (2016) further compared the differences among Hippocampus-fed Artemia, frozen Artemia, and Mysis. The results showed that the crude protein content of the frozen Artemia group was the lowest among the three groups. The content of polyunsaturated fatty acids in the Artemia group was the highest, while that in the Mysis group was the lowest. Frozen bait may not fully meet the nutritional needs of Hippocampus. In addition, the Mysis group had the most abundant nucleosides, and thymine was not detected in the frozen Mysis group or the Artemia group (Yan Z. Z., 2019). In the selection of baits, mixing of different baits may meet different nutritional needs.
Extraction Strategies of Hippocampus
Extraction is a method of obtaining effective ingredients from medicinal materials through chemical processes, such as solvent treatment, or by mechanical processes, such as supercritical fluid extraction and microwave-assisted extraction (Chaves et al., 2020). Extraction of Hippocampus active ingredients is the basis for the study of its pharmacological activity. The potential targets and mechanisms of action of Hippocampus in disease treatment must be clarified. Extraction methods for different ingredients should be further researched to find a high-efficiency method that presents a shorter extraction time.
Extraction Strategies of Hydrophilic Ingredients
The hydrophilic ingredients of Hippocampus are mainly amino acids, peptides, glycoproteins, nucleosides, etc., Chen et al. (1997) compared amino acids in water and ethanol extracts of Hippocampus. The results showed that the Hippocampus water extract contained 17 types of amino acids, while the ethanol extract contained only 10 types of amino acids. The essential amino acid content of the Hippocampus water extract was also higher than that of the ethanol extract. Hippocampus peptides were obtained by enzyme hydrolysis and chromatography technologies. For bioactive peptides from marine organisms, the first step was the trituration of the tissues, followed by initial treatment (organic extraction, concentration, and/or partitioning). Under the guidance of activity assays, chromatographic technology was used for prepurification, and purified peptides were finally obtained by high-performance liquid chromatography using reversed-phase (RP-HPLC). Alternatively, after tissue separation, enzymatic hydrolysis could be employed to identify bioactive peptides (Macedo et al., 2021). Enzymatic hydrolysis is a commonly used method to extract Hippocampus peptides. Commonly selected enzymes include pronase E, alcalase, protamex, trypsin, papain, and pepsin (Table 1). Enzymes and substrates at a ratio of 1:100 (w/w) were mixed under certain conditions, and then the mixture was inactivated at 100°C for 10 min and stored at −80°C (Pangestuti et al., 2013). In addition, Hippocampus was defatted with ethyl acetate or CO2 supercritical fluid extraction technology and then mixed with enzyme (Jiang et al., 2014). Jiang et al. (2014) studied the condition of compound enzyme hydrolysis and found that the hydrolysis rate of alcalase and trypsin was 2–3% higher than that of single enzyme hydrolysis. We summarized the enzyme reaction under different conditions to obtain optimized reaction conditions (Table 1). Enzymes were selected according to the different needs of research. For example, Hippocampus trimaculatus Leach protein was hydrolyzed by four different proteases (trypsin, pepsin, papain, and alcalase) to prepare peptides, and, compared with other hydrolysates, alcalase hydrolysates exhibited the highest ACE inhibitory activity (Xu, 2015). Nucleosides of Hippocampus are generally extracted with 50% ethanol or water, followed by HPLC analysis (Wei et al., 2015; Yan Z. Z., 2019). Chen et al. (2010) developed an online rapid method for the identification of hypoxanthine, and the antioxidant hypoxanthine was identified from a water extract of Hippocampus japonicus Kaup by HPLC-ESI-TOF/MS combined with an ABTS free radical scavenging online detection system. Zheng et al. (2012) used different antioxidant evaluation experiments for DPPH free radical scavenging, and an online detection system also identified hypoxanthine from water extracts of Hippocampus kuda Bleeker and Hippocampus japonicus Kaup. The online detection system combines biological activity and chemical separation, and the target ingredient may be quickly separated.
Extraction Strategies of Hydrophobic Ingredients
More reports have focused on Hippocampus hydrophobic ingredients than other types, and these hydrophobic ingredients include fatty acids, steroids, and phospholipids. The extraction of fatty acids in Hippocampus is performed by the traditional solvent extraction method. Nicolás et al. (2014) directly soaked Hippocampus powder in chloroform:methanol (2:1) for 24 h to extract total lipids. The extraction solvent of chloroform:methanol (2:1) was also used for ultrasonic extraction (Xu et al., 1994). The results showed that there were more types of fatty acids in the ultrasonic extraction. Extraction methods using ether as the extraction solvent include Soxhlet extraction (Zhang et al., 1997), rapid fat extraction (Zhang and Wang, 1996), and ultrasonic extraction (Chen, 2015). Among them, the rapid fat extraction method greatly shortened the extraction period and effectively reduced the oxidation of Hippocampus unsaturated fatty acids (Zhang and Wang, 1996). In summary, ultrasonic extraction and rapid fat extraction are generally used in the extraction of fatty acids. Compared with traditional solvent extraction methods with the problems of solvent residue and heating damage to fatty acids, CO2 supercritical fluid extraction can extract total lipids at low temperatures and without organic solvents (Jiang et al., 2013). Huang and Xu (2016) compared the effects of chloroform-methanol extraction, absolute ethanol extraction, petroleum ether Soxhlet extraction, and CO2 supercritical fluid extraction on the lipid and fatty acid constituents of Hippocampus. The results showed that the types of fatty acids by the four methods were almost the same while the contents of unsaturated fatty acids in chloroform-methanol extraction and absolute ethanol extraction were the highest, with saturated fatty acids in CO2 supercritical fluid extraction the highest. The extraction of Hippocampus steroids has generally been performed by ethanol extraction followed by organic solvent (n-butanol, ethyl acetate, and petroleum ether) extraction. The fractions were then repeatedly chromatographed with silica gel column chromatography using a stepwise gradient elution of different solvents (Wu et al., 2017b). The total lipids of the Hippocampus were extracted by chloroform-methanol (2:1), 75% ethanol, and water, and the content of total phospholipids was identified by molybdenum blue colorimetry. Studies have shown that the chloroform-methanol (2:1) extraction method has the highest content of total phospholipids (Xu et al., 1994; Sheng et al., 2011).
Pharmacological Activities of Hippocampus
The pharmacological activities of Hippocampus were sorted according to the different extraction methods (enzymolysis, alcohol extraction, water extraction, and other extraction methods). Modern pharmacological studies have revealed that Hippocampus shows various biological activities, such as sex hormone-like effects and antioxidation, anti-inflammation, antitumor effects, neuroprotection, and antimicrobial activities. Different extraction portions of Hippocampus exhibited particular pharmacological activities, which may be related to the ingredient types. For example, the antihypertensive and neuroprotective activities of enzymolysis portions were based on the peptides extracted. The alcohol extraction portions mainly showed sex hormone-like effects and anti-inflammation, which were closely related to steroids, ketones, etc. In Figure 6, the extraction methods, ingredients, and pharmacological activities are summarized.
Enzymolysis
The pharmacological activities of Hippocampus peptides include antihypertensive, antioxidation, and anti-inflammation activities (Figure 6). The ACE inhibitory peptide obtained from Hippocampus abdominalis Lesson induced an increase in NO content in blood vessels through the expression of phosphor-endothelial nitric oxide synthase (p-eNOS) to promote vasodilation and lower blood pressure (Je et al., 2020). ACE is part of the renin-angiotensin system and plays a vital role in regulating blood pressure, and ACE inhibitors are effective in the treatment of hypertension (Li et al., 2014). In addition, free radicals are in a special state of balance in the human body, and when this balance is disrupted, they may cause oxidative stress, which can lead to the damage of blood vessels and cardiovascular diseases. Interestingly, Gu and Xu (2016) found that Hippocampus ACE inhibitory peptide showed DPPH radical scavenging activity. One of the medicinal candidates for the treatment of hypertension may be bifunctional peptides with both ACE inhibitory and antioxidant activity. The therapeutic effects of peptide SHP-1 isolated from Hippocampus kuda Bleeker on arthritis were observed with human chondrocyte-like SW-1353 and human osteoblast-like MG-63 cells. The inhibition rates of SHP-1 on collagen release in SW-1353 and MG-63 cells were 40.35–59.96% and 60.82–85.82%, respectively. SHP-1 inhibited the expression of collagenase 1,13 and NO induced by the proinflammatory cytokine 12-O-tetradecanoylphorbol-13-acetate (TPA) and blocked NF-KB/p38 kinase (Ryu et al., 2010). Ryu (2010) further isolated a Hippocampus peptide with alkaline phosphatase activity and showed that it not only promoted the differentiation of MG-63 osteoblasts and SW-1353 chondrocytes but also inhibited the expression of the inflammatory factors cyclooxygenase-2 (COX-2) and inducible nitric oxide synthase (iNOS). A peptide showed remarkable anti-inflammatory activity by regulating the NF-κB and MAPK signaling pathways. Patients with arthritis have persistent joint swelling and restricted movement due to pain (Mandl, 2019). The anti-inflammatory effect of peptides may be associated with the traditional efficacy of Hippocampus in reducing swelling.
Hippocampus also presents an anti-fatigue effect (Hu et al., 2000). Kang et al. (2017) found that enzymatic hydrolysates of Hippocampus abdominalis Lesson promoted the proliferation of C2C12 myoblasts and increased the contents of physical activity markers, such as ATP and glycogen. Hippocampus enzymatic hydrolysates not only promoted the elimination of lactic acid and urea nitrogen during exercise but also strengthened the antifatigue activity with increasing exercise intensity. Compared with the antifatigue effect of the one-time fatigue model, the incremental exercise fatigue model was more effective. For normal mice, Hippocampus enzymatic hydrolysates improved the survival time under hypoxia and cold conditions (Peng and Chen, 2005). Accordingly, supplementation with Hippocampus enzymatic hydrolysates may be a natural health product that has an improved effect on the human body. For nervous diseases, Hippocampus enzymatic hydrolysates promoted the recovery of neurological function and reduced the volume of cerebral infarction and brain edema in animal models of ischemia-reperfusion brain damage (Feng et al., 2005). The peptide HTP-1 had a protective effect on Aβ42-induced PC12 cells and may be an active ingredient of Hippocampus for the treatment of neurodegenerative disease (Pangestuti et al., 2013). However, the neuroprotective mechanisms of Hippocampus require further investigation. In a coculture system with PC12 cells and BV-2 cells induced by Aβ42, HTP-1-protected PC12 cells were not subjected to the neurotoxicity of BV-2 cells. HTP-1 attenuated the neurotoxic mediators released by BV-2 cells and activated PI3K/Akt by inducing transforming growth factor-β (TGF-β), which is the main signaling pathway of neuronal survival (Pangestuti and Kim, 2015).
Alcohol Extraction
The Hippocampus activity of warming and tonifying kidney yang was closely related to the alcohol extract of Hippocampus. Chen et al. (2009) studied the effect of the n-butanol extract from Hippocampus on a mouse model of kidney yang deficiency. After 15 days of continuous administration, the results showed that the n-butanol group improved the rectal temperature, grip strength, testis index, and seminal vesicle index of the model mouse. The ethanol extract of Hippocampus was orally administered to normal and cyclophosphamide model mice, and it was found to increase the overall sperm number and viability. From the perspective of modern science, Hippocampus improves the symptoms of kidney deficiency, such as weakness at the waist and knees, frigidity, chilliness, and cold limbs (Zhang et al., 1995). The total sterol content in Hippocampus kuda Bleeker not only increased the content of testosterone in vivo but also changed the structure of the seminiferous duct and promoted the formation of sperm. However, long-term high-dose intake of total sterols might change the shape of the kidney and testis (Sun, 2015). Over thousands of years, traditional Chinese clinical practices have supported the effect of Hippocampus on improving sexual function, although the material basis and concrete mechanism of efficacy were not clear. The enzymatic hydrolysate of Hippocampus had a certain therapeutic effect on arthritis, while the alcohol extract was more effective in the treatment of neuroinflammation. Himaya et al. (2011, 2012) isolated 1-(5-bromo-2-hydroxy-4-methoxyphenyl)ethanone (SE1) and paeonol from Hippocampus kuda Bleeker and showed that it inhibited the expression of proinflammatory cytokines in BV-2 microglial cells induced by lipopolysaccharide (LPS). This compound played an anti-inflammatory role by inhibiting the phosphorylation of JNK and p38 in the MAPK pathway and nuclear heterotrophs in the NF-kB pathway (Figure 7). Yan et al. (2019) further found that SE1 and paeonol improved the morphology of activated microglia with enlarged cell bodies and shorter synapses. Recent studies have shown that neurodegenerative diseases are closely related to neuroinflammation caused by activated microglia (Sarlus and Heneka, 2017). SE1 and paeonol extracted from Hippocampus-modulated neuroinflammation and may be potential candidates for treating neurodegenerative diseases, such as Alzheimer’s disease (AD). To verify the effects and mechanisms of SE1 and paeonol on AD, in vitro or in vivo experiments with pharmacological approaches are needed in the future. Recently, patients with schizophrenia have been shown to have an increased inflammatory response, and autophagy dysfunction caused by inflammation might continue to increase the inflammatory response. Studies have shown that the alcohol extract of Hippocampus kelloggi Jordan et Snyder exerts anti-inflammatory effects by improving autophagy, thus revealing a new possible target for Hippocampus-based therapeutics to combat schizophrenia (Yan L., 2019). In addition, the methanol extract of Hippocampus increased the concentration of neurotransmitters and the expression of brain-derived neurotransmitters by inhibiting the secretion of proinflammatory factor interleukin-1β (1L-1β), which improved depressive behavior in mice and restored normal neurotransmitter function (Li et al., 2020). The Hippocampus anti-inflammatory effect is associated with the regulation of the MAPK and NF-κB pathways, which are primarily associated with inflammation (Wu et al., 2017a). Wu et al. (2020) identified miR-98-5p from the cellular RNA library, and 3β-hydroxycholest-5-en-7-one isolated from Hippocampus regulated the expression of the miR-98-5p target gene TNFAIP3 to inhibit the excessive secretion of proinflammatory factors and produce an anti-inflammatory effect. The screening of antitumor active ingredients from TCM has become a hot field. Studies have shown that the alcohol extract of Hippocampus has an inhibitory effect on tumor cells. In a study of dihydrotestosterone-induced LNCaP cells of prostate cancer, dual-targeting AKT and AR signaling showed that brassicasterol from Hippocampus abdominalis Lesson exerted an anticancer effect (Xu et al., 2020). Sphingoid bases were purified from Hippocampus using lipid extraction, saponification, and acid hydrolysis (Yu, 2018). The IC50 of human chronic myeloid leukemia cells treated with sphingoid bases for 24 h was 37.17 μg/ml. Further investigation of the mechanisms by Western blot and RT–PCR analysis revealed that sphingoid bases exerted their antitumor activity by inducing apoptosis via both the mitochondrial apoptosis pathway and the death receptor pathway. In addition, sphingoid bases also inhibited the formation of neovascularization and promoted cell apoptosis by inhibiting the expression of COX-2. The antitumor activity of Hippocampus is mainly caused by low- and medium-polarity ingredients and has great research prospects in cancer therapy.
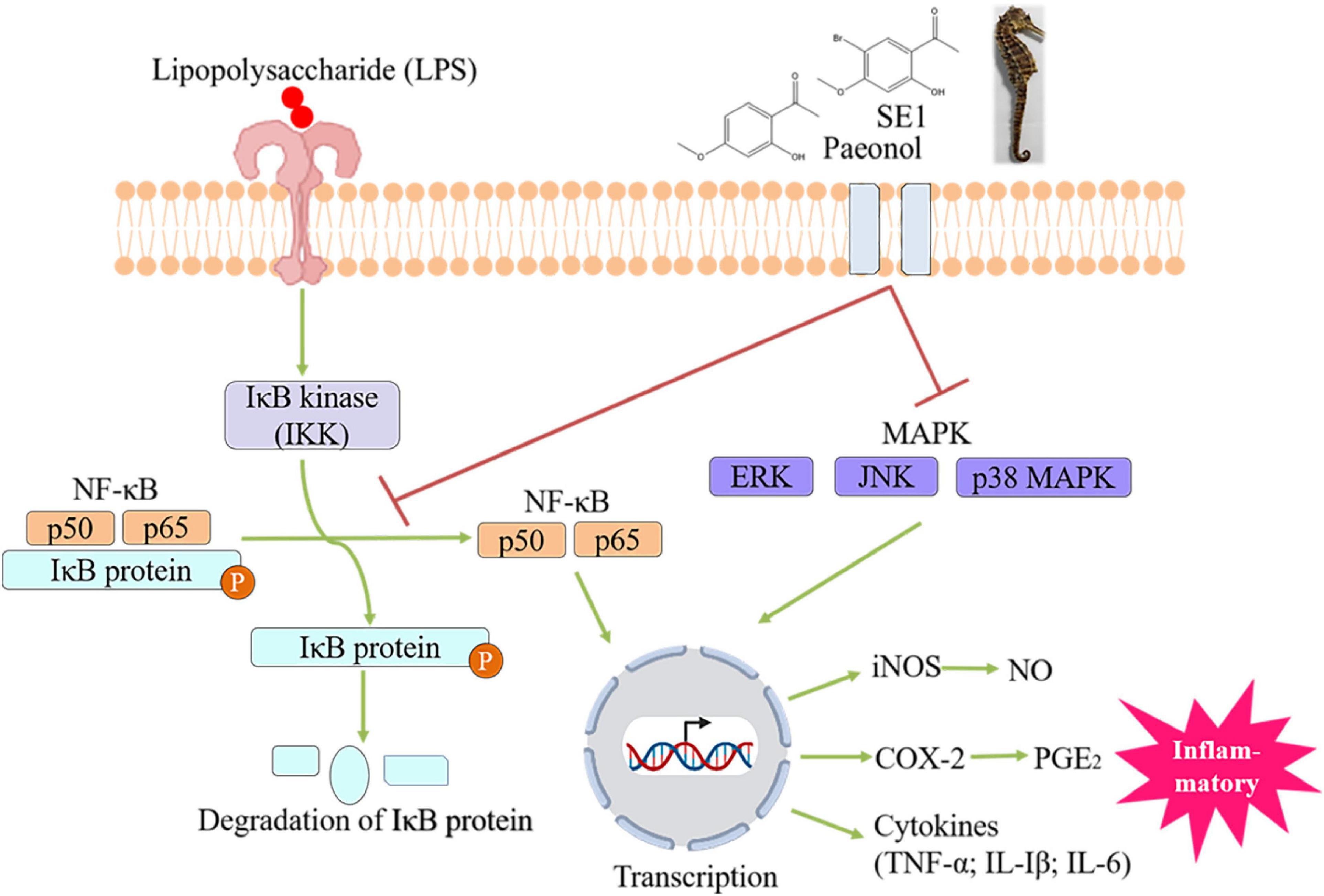
Figure 7. Paeonol and 1-(5-bromo-2-hydroxy-4-methoxyphenyl)ethanone(SE1) from Hippocampus Kuda Bleeker blocked the LPS-stimulated inflammatory responses in BV-2 microglial cell via modulating MAPK and NF-κB-signaling pathways.
The antioxidant activity of Hippocampus was also explored. Three phthalic acid derivatives isolated by Qian et al. (2012) from the methanol extract of Hippocampus kuda Bleeker had antioxidant activity, among which 2,12-diethyl-11-methylhexadecyl 2-ethyl-11-methylhexadecylphthalate had the strongest antioxidant activity and exhibited dose-dependent effects; moreover, it is a safe and effective antioxidant that inhibited reactive oxygen in mouse macrophages (RAW264.7) and had no toxic effect on cells. The ethanol extract of Hippocampus had a certain immunomodulatory effect. On the one hand, it increased the spleen weight of mice and promoted lymphocyte proliferation and phagocyte function (Chen et al., 1995). On the other hand, it inhibited the delayed-type hypersensitivity induced by dinitrochlorobenzene in mice (Zhu, 2005). Brain monoamine oxidase B (MAO-B) increases during aging, which is considered a sign of aging. The alcohol extract of Hippocampus Kuda Bleeker had a significant inhibitory effect on MAO-B activity in the mouse brain, suggesting that the Hippocampus delayed aging (She et al., 1995). The methanol extract of Hippocampus had an obvious inhibitory effect on the experimental common carotid artery and cerebral thrombosis in mice. The inhibition rate of 200 mg/kg extract on cerebral thrombosis was 46.8%. The main active ingredients were unsaturated fatty acids, and the content of 9,12-octadecadienoic acid accounted for 18.5% (Xu and Xu, 1997). In addition, the ethanol extracts from five species of Hippocampus promoted the differentiation of osteoblasts and the formation of bone nodules and had a certain therapeutic effect on osteoporosis (Wang, 2015).
Water Extraction
Antioxidant activity was found in both the water extracts of Hippocampus japonicus Kaup and Hippocampus Kuda Bleeker (Chen et al., 2010; Zheng et al., 2012), suggesting that the water extract of Hippocampus had antioxidant activities. The most important key factor affecting the degree of pharmacological activity was the extraction time, followed by the extraction temperature (Yi et al., 2006). Qian et al. (2008) compared the antioxidant activities of the methanol extract, ethanol extract, and water extract. The antioxidant activity degree of the methanol extract was higher than that of the other two extracts, and its activity degree was between that of α-tocopherol and vitamin C. In general, moderately and less hydrophilic ingredients showed strong antioxidant activity. The warming and tonifying kidney yang effect has been associated with the alcohol extract of Hippocampus. However, the water extract of Hippocampus also improved the ability of the testicles in the mice model with kidney yang deficiency, and the testis index was higher than that observed for the petroleum ether extract at the same dose (Chen et al., 2009).
Other Extraction Methods
Sun et al. (2012) synthesized the antimicrobial peptide HKPLP from the brooding pouch cDNA library of Hippocampus kuda Bleeker. The results indicated that the most sensitive bacterium of HKPLP was Staphylococcus aureus. In addition, HKPLP appeared to have a minor inhibitory effect on fungi. Ko et al. (2016) identified a goose-type lysozyme (ShLysG) from Hippocampus abdominalis Lesson that had a certain inhibitory effect against gram-positive/negative bacteria and a potential immune-protecting effect against pathogens. Bacterial pathogen factors are one of the important factors affecting the survival of marine organisms. Isolation of marine microorganisms with antimicrobial activity from the intestinal contents and skin mucus of Hippocampus guttulatus Cuvier promoted the development of breeding and rearing of Hippocampus (Balcazar et al., 2010). In addition, the ethyl acetate extract from Hippocampus significantly improved benign prostatic hyperplasia in castration and testosterone mouse models (Xu et al., 2014).
Conclusion
The increasing demand for safe and effective medicines has led to increased attention on marine natural products. This review mainly focused on the ingredients, extraction methods, pharmacological activities, and traditional prescriptions containing different species of Hippocampus. Hippocampus is mainly distributed in the Pacific region, with the largest species number in Australia. Hippocampus trade in China and surrounding Southeast Asian countries is high. The active ingredients of Hippocampus include hydrophobic ingredients, such as steroids and fatty acids, and hydrophilic ingredients, such as peptides and glycoproteins. Studies have shown that anti-inflammation, antioxidation, antitumor, and neuroprotective effects are the important key functions of Hippocampus. The alcohol extract of Hippocampus not only warms and tonifies kidney yang but also blocks neuroinflammation by regulating the MAPK and NF-κB pathways. In particular, Hippocampus peptides reduce the toxicity of Aβ42 to nerve cells. The neuroprotective activity of Hippocampus indicates its ability to treat nervous system diseases. In addition, an animal experiment involving traditional prescriptions containing Hippocampus also showed its antitumor value and treatment of benign prostatic hyperplasia. In the future, quality evaluation standards for Hippocampus should be established, and the targets and mechanisms of the pharmacological activity, active ingredients, and pharmacological activities of Hippocampus need to be further studied. The kidney-brain axis may be the key point at which Hippocampus impacts neurodegenerative diseases, and Hippocampus peptides may have broader application prospects.
Author Contributions
XL, XF, and XC conceived and proposed the idea, designed the study, and prepared the manuscript. XZ contributed to Supplementary Table 6. JL contributed to Figure 4. XF was the project organization leader. XL and XF guided the manuscript writing. All authors contributed to the article and approved the submitted version.
Funding
This work was supported by the National High Technology Research and Development Program of China (863 Program) (No. 2013AA093001).
Conflict of Interest
The authors declare that the research was conducted in the absence of any commercial or financial relationships that could be construed as a potential conflict of interest.
Publisher’s Note
All claims expressed in this article are solely those of the authors and do not necessarily represent those of their affiliated organizations, or those of the publisher, the editors and the reviewers. Any product that may be evaluated in this article, or claim that may be made by its manufacturer, is not guaranteed or endorsed by the publisher.
Supplementary Material
The Supplementary Material for this article can be found online at: https://www.frontiersin.org/articles/10.3389/fmars.2021.774927/full#supplementary-material
References
Althagbi, H. I., Alarif, W. M., Al-Footy, K. O., and Abdel-Lateff, A. (2020). Marine-derived macrocyclic alkaloids (MDMAs): chemical and biological diversity. Mar. Drugs 18:368. doi: 10.3390/md18070368
Balcazar, J. L., Loureiro, S., Da Silva, Y. J., Pintado, J., and Planas, M. (2010). Identification and characterization of bacteria with antibacterial activities isolated from seahorses (Hippocampus guttulatus). J. Antibiot. (Tokyo) 63, 271–274. doi: 10.1038/ja.2010.27
Blunt, J. W., Carroll, A. R., Copp, B. R., Davis, R. A., Keyzers, R. A., and Prinsep, M. R. (2018). Marine natural products. Nat. Prod. Rep. 35, 8–53. doi: 10.1039/c7np00052a
Bohic, S., Ghersi-Egea, J. F., Gibon, J., Paoletti, P., Arnaud, J., Hunot, S., et al. (2011). [Biological roles of trace elements in the brain with special focus on Zn and Fe]. Rev. Neurol. (Paris) 167, 269–279. doi: 10.1016/j.neurol.2010.07.035
Carroll, A. R., Copp, B. R., Davis, R. A., Keyzers, R. A., and Prinsep, M. R. (2019). Marine natural products. Nat. Prod. Rep. 36, 122–173. doi: 10.1039/c8np00092a
Carroll, A. R., Copp, B. R., Davis, R. A., Keyzers, R. A., and Prinsep, M. R. (2020). Marine natural products. Nat. Prod. Rep. 37, 175–223. doi: 10.1039/c9np00069k
Carroll, A. R., Copp, B. R., Davis, R. A., Keyzers, R. A., and Prinsep, M. R. (2021). Marine natural products. Nat. Prod. Rep. 38, 362–413. doi: 10.1039/d0np00089b
Casey, S. P., Hall, H. J., Stanley, H. F., and Vincent, A. C. (2004). The origin and evolution of seahorses (genus Hippocampus): a phylogenetic study using the cytochrome b gene of mitochondrial DNA. Mol. Phylog. Evol. 30, 261–272. doi: 10.1016/j.ympev.2003.08.018
Chaves, J. O., de Souza, M. C., da Silva, L. C., Lachos-Perez, D., Torres-Mayanga, P. C., Machado, A., et al. (2020). Extraction of flavonoids from natural sources using modern techniques. Front. Chem. 8:507887. doi: 10.3389/fchem.2020.507887
Chen, C. Q. (1983). A Supplement to Materia Medica. Wuhu: Academic Research of Wannan Medical College.
Chen, D., Chen, J., Gao, J., and Cao, X. Y. (2018). Evaluation of the antioxidant activity of Hippocampus erectus peptides. Food R&D 39, 10–15. doi: 10.3969/j.issn.1005-6521.2018.08.002
Chen, J., Zhao, H., Shi, Q., Zhang, D., Cheng, H., Wang, X., et al. (2010). Rapid screening and identification of the antioxidants in Hippocampus japonicus Kaup by HPLC-ESI-TOF/MS and on-line ABTS free radical scavenging assay. J. Sep. Sci. 33, 672–677. doi: 10.1002/jssc.200900544
Chen, L. (2015). Study on Identification and Quality Standard of Hippocampus. Master’s thesis. Shanghai: The Second Military Medical University.
Chen, L., Wang, X., and Huang, B. (2015). The genus Hippocampus–a review on traditional medicinal uses, chemical constituents and pharmacological properties. J. Ethnopharmacol. 162, 104–111. doi: 10.1016/j.jep.2014.12.032
Chen, S. H., Lv, G. Y., Fan, J., Yan, M. Q., Ye, H., Fang, Z., et al. (2009). Effects of different extracts from Hippocampus japonicus on kidney-yang deficieny mice induced by extradiol. China Tradit. Herb. Drugs 40, 258–262.
Chen, W. N., Xu, L. Z., Gao, E., Li, H. Z., and Miao, N. F. (1995). The pharmacological effects of extracts of hippocampus. Acta Acad. Med. Weifang 17, 105–109.
Chen, W. N., Yu, S. M., Li, Y. H., Xu, L. Z., and Zhang, W. (1997). Assaying of amino acids and trace elements in the extract of hippocampUS. Acta Acad. Med. Weifang 19, 25–26. doi: 10.1530/eje.0.1360316
Chen, X., Yi, Y., You, X., Liu, J., and Shi, Q. (2019). High-throughput identification of putative antimicrobial peptides from multi-omics data of the lined seahorse (Hippocampus erectus). Mar. Drugs 18:10030. doi: 10.3390/md18010030
Chinese, P. C. (2020). Pharmacopoeia of the People’s Republic of China. Beijing: China Medical Science Press.
Choi, Y.-U., Rho, S., Park, H.-S., and Kang, D.-H. (2012). Population characteristics of two seahorses, Hippocampus coronatus and Hippocampus mohnikei, around seagrass beds in the southern coastal waters of Korea. Ichthyol. Res. 59, 235–241.
Choo, C. K., and Liew, H. C. (2003). Spatial distribution, substrate assemblages and size composition of sea horses (Family Syngnathidae) in the coastal waters of Peninsular Malaysia. J. Mar. Biol. Assoc. U. K. 83, 271–276. doi: 10.1017/S0025315403007069h
CITES (2021b). Hippocampus [Online]. Available online at: https://checklist.cites.org/#/en (accessed September 10, 2021).
CITES (2021a). Hippocampus [Online]. Available online at: https://trade.cites.org/ (accessed January 20, 2021).
Conte, M., Fontana, E., Nebbioso, A., and Altucci, L. (2021). Marine-derived secondary metabolites as promising epigenetic bio-compounds for anticancer therapy. Mar. drugs 19:15. doi: 10.3390/md19010015
Convention on International Trade in Endangered Species of Wild Fauna and Flora (2020). Available online at: https://trade.cites.org/ (accessed December 18, 2020).
Dayanidhi, D. L., Thomas, B. C., Osterberg, J. S., Vuong, M., Vargas, G., Kwartler, S. K., et al. (2021). Exploring the diversity of the marine environment for new anti-cancer compounds. Front. Mar. Sci. 7:1184. doi: 10.3389/fmars.2020.614766
De Jong, W. H., and Borm, P. J. (2008). Drug delivery and nanoparticles:applications and hazards. Int. J. Nanomed. 3, 133–149. doi: 10.2147/ijn.s596
Ermolenko, E. V., Imbs, A. B., Gloriozova, T. A., Poroikov, V. V., Sikorskaya, T. V., and Dembitsky, V. M. (2020). Chemical diversity of soft coral steroids and their pharmacological activities. Mar. Drugs 18:613. doi: 10.3390/md18120613
Faleiro, F., and Narciso, L. (2010). Lipid dynamics during early development of Hippocampus guttulatus seahorses: searching for clues on fatty acid requirements. Aquaculture 307, 56–64. doi: 10.1016/j.aquaculture.2010.07.005
Fan, G. (2012). Application of 1H NMR-Based Metabolomics for the Species Differentiation and Quality Evaluation of Traditional Chinese Medicine and Tibetan Medicine From Various Species. Dissertation’s thesis. Chengdu: Chengdu University of Traditional Chinese Medicine.
Feng, X., Wu, Z. F., Yang, L., Zhou, Y., Wang, Q., Liu, Y. Z., et al. (2005). Effects of haima extraction on experimental ischemia/reperfusion brain damage. J. Hunan Norm. Univ. (Med. Sci.) 2, 1–4.
FishBase (2021). Hippocmpus [Online]. Available online at: https://www.fishbase.se/Nomenclature/ValidNameList.php?syng=Hippocampus&syns=&vtitle=Scientific+Names+where+Genus+Equals+%3Ci%3EHippocampus%3C%2Fi%3E&crit2=CONTAINS&crit1=EQUAL (accessed September 10, 2021).
Foster, S. J., and Vincent, A. C. J. (2004). Life history and ecology of seahorses: implications for conservation and management. J. Fish. Biol. 65, 1–61. doi: 10.1111/j.0022-1112.2004.00429.x
Ge, Y. Q., Zhu, L. Y., Chen, M., Zhang, G. J., Huang, Z., and Cheng, R. B. (2018). Complete mitochondrial genome sequence for the endangered Knysna seahorse Hippocampus capensis Boulenger 1900. Conserv Genet Resour 10, 461–465. doi: 10.1007/s12686-017-0849-3
Gerardo, B., Cabral Pinto, M., Nogueira, J., Pinto, P., Almeida, A., Pinto, E., et al. (2020). Associations between Trace Elements and cognitive decline: an exploratory 5-year follow-up study of an elderly cohort. Int. J. Environ. Res. Public Health 17:6051. doi: 10.3390/ijerph17176051
Gu, W., and Xu, Y. J. (2016). Preparation of Hippocampus ACE inhibitory peptide and determination of antioxidant capacity. Sci. Technol. Food Industry 37, 201–206. doi: 10.13386/j.issn1002-0306.2016.05.031
Han, F. J. (2002). The mechanism study on hai-ma-sheng-sui pill resisting the tumor on S180 and SKOV3 Mice. J. Harb. Univ. Comm. Natur. Sci. 18, 66–68.
Han, S. L. (2013). Taxonomy, Resources, Utilization and Conservation of Sea Horses in China. Master’s thesis. Guangxi: Guangxi Normal University.
Himaya, S. W., Ryu, B., Qian, Z. J., Li, Y., and Kim, S. K. (2011). 1-(5-bromo-2-hydroxy-4-methoxyphenyl)ethanone [SE1] suppresses pro-inflammatory responses by blocking NF-kappaB and MAPK signaling pathways in activated microglia. Eur. J. Pharmacol. 670, 608–616. doi: 10.1016/j.ejphar.2011.09.013
Himaya, W. A., Ryu, B. M., Qian, Z. J., and Kim, S. K. (2012). Paeonol from Hippocampus kuda bleeler suppressed the neuro-inflammatory responses in vitro via NF-κB and MAPK signaling pathway. Toxicol. in Vitro 26, 878–887. doi: 10.1016/j.tiv.2012.04.022
Hosseini, B., Nourmohamadi, M., Hajipour, S., Taghizadeh, M., Asemi, Z., Keshavarz, S. A., et al. (2019). The effect of omega-3 fatty acids, EPA, and/or DHA on male infertility: a systematic review and meta-analysis. J. Diet. Suppl. 16, 245–256. doi: 10.1080/19390211.2018.1431753
Hou, F., Wen, L., Peng, C., and Guo, J. (2016). Identification of marine traditional Chinese medicine dried seahorses in the traditional Chinese medicine market using DNA barcoding. Mitochon. Dna Dna Mapp. Seq. Anal. 16, 1–6. doi: 10.1080/24701394.2016.1248430
Hu, J. Y., Li, B. F., Li, Z. J., and lv, P. (2000). An experimental study on anti-fatigue effects of eight marine pharmakons. Chin. J. Mar. Drugs 2, 56–58.
Huang, B. Q., Ai, C. R., and Mai, Q. F. (1997). The preparation of compound sea-horse medicinal liquor and its health protection effect. Chin. J. Mar. Drugs 4, 44–49.
Huang, D., and Xu, Y. J. (2016). Different extraction process for Hippocampus kuda lipid and its analysis of the fatty acid composition. Chin. J. Mar. Drugs 35, 35–40. doi: 10.13400/j.cnki.cjmd.2016.02.007
Huang, R. M., Chen, Y. N., Zeng, Z. Y., Gao, C. H., Su, X. D., and Peng, Y. (2014). Marine nucleosides: structure, bioactivity, synthesis and biosynthesis. Mar. Drugs 12, 5817–5838. doi: 10.3390/md12125817
IUCN (2021). Hippocampus [Online]. Available online at: https://www.iucnredlist.org/search?query=Hippocampus&searchType=species (accessed September 10, 2021).
Je, J.-G., Kim, H.-S., Lee, H.-G., Oh, J.-Y., Lu, Y. A., Wang, L., et al. (2020). Low-molecular weight peptides isolated from seahorse (Hippocampus abdominalis) improve vasodilation via inhibition of angiotensin-converting enzyme in vivo and in vitro. Process Biochem. 95, 30–35. doi: 10.1016/j.procbio.2020.04.016
Ji, W. T., and Yu, X. (2020). A reinterpretation of yin-yang theory of TCM based on chaos theory. Liaoning J. Tradit. Chin. Med. 47, 77–79.
Jia, W., Gao, W. Y., Wang, T., Liu, Y. B., Xue, J., and Xiao, P. G. (2003). Cataplasma of traditional Chinese medicine. Chin. J. Chin. Mat. Med. 28, 7–11.
Jia, Y. Y., and Yao, Q. Y. (1990). Comparative analysis of trace elements and amino-acids in Hippocampus kuda bleeker and H.parvus. Chin. J. Mar. Drugs 9, 13–15.
Jiang, Z. Z., Xu, Y. J., and Su, Y. T. (2013). The extraction technology of fat from bone powder of sea horse by sfe and the analysis of fatty acid composition. Chin. J. Mar. Drugs 32, 47–52. doi: 10.13400/j.cnki.cjmd.2013.02.009
Jiang, Z., Xu, Y., and Su, Y. (2014). Preparation process of active enzymolysis polypeptides from seahorse bone meal. Food Sci. Nutr. 2, 490–499. doi: 10.1002/fsn3.125
Kang, N., Kim, S.-Y., Rho, S., Ko, J.-Y., and Jeon, Y.-J. (2017). Anti-fatigue activity of a mixture of seahorse (Hippocampus abdominalis) hydrolysate and red ginseng. Fish Aquat. Sci. 20:3. doi: 10.1186/s41240-017-0048-x
Khalifa, S. A. M., Elias, N., Farag, M. A., Chen, L., Saeed, A., Hegazy, M. F., et al. (2019). Marine natural products: a source of novel anticancer drugs. Mar. Drugs 17:491. doi: 10.3390/md17090491
Ko, J., Wan, Q., Bathige, S., and Lee, J. (2016). Molecular characterization, transcriptional profiling, and antibacterial potential of G-type lysozyme from seahorse (Hippocampus abdominalis). Fish Shellf. Immunol. 58, 622–630. doi: 10.1016/j.fsi.2016.10.014
Koldewey, H. J., and Martin-Smith, K. M. (2010). A global review of seahorse aquaculture. Aquaculture 302, 131–152. doi: 10.1016/j.aquaculture.2009.11.010
Kumaravel, K., Ravichandran, S., Balasubramanian, T., and Sonneschein, L. (2012). Seahorses – a source of traditional medicine. Nat. Prod. Res. 26, 2330–2334. doi: 10.1080/14786419.2012.662650
Li, E. C., Heran, B. S., and Wright, J. M. (2014). Angiotensin converting enzyme (ACE) inhibitors versus angiotensin receptor blockers for primary hypertension. Cochrane Database Syst. Rev. 2014:CD009096. doi: 10.1002/14651858.CD009096.pub2
Li, K. W., Yan, L., Zhang, Y. P., Yang, Z. Y., Zhang, C., Li, Y. J., et al. (2020). Seahorse treatment improves depression-like behavior in mice exposed to CUMS through reducing inflammation/oxidants and restoring neurotransmitter and neurotrophin function. J. Ethnopharmacol. 250:112487. doi: 10.1016/j.jep.2019.112487
Li, Y., Qian, Z. J., and Kim, S. K. (2008). Cathepsin B inhibitory activities of three new phthalate derivatives isolated from seahorse, Hippocampus kuda bleeler. Bioorg. Med. Chem. Lett. 18, 6130–6134. doi: 10.1016/j.bmcl.2008.10.016
Liang, B., Cai, X.-Y., and Gu, N. (2021). Marine natural products and coronary artery disease. Front. Cardiovas. Med. 8:1135. doi: 10.3389/fcvm.2021.739932
Lin, Q., Lin, J. D., and Wang, C. (2009). Biochemical composition of the wild and cultured seahorses, Hippocampus kuda bleeker and Hippocampus trimaculatus leach. Aquac. Res. 40, 710–719. doi: 10.1111/j.1365-2109.2008.02149.x
Lin, Q., Lin, J. D., Lu, J. Y., and Li, B. J. (2008). Biochemical composition of six seahorse species, Hippocampus sp., from the Chinese coast. J. World Aquacult. Soc. 39, 225–234. doi: 10.1111/j.1749-7345.2008.00159.x
Liu, F. Y., Yuan, Y., Jin, Y., Qin, W., Jiang, C., Zhao, Y. Y., et al. (2018). Multiplex PCR to simultaneous identification of five traditional Chinese medicinal seahorses. Chin. J. Chin. Mater. Med. 43, 4562–4568. doi: 10.19540/j.cnki.cjcmm.20181025.012
Liu, X., and Lin, Y. (2006). Quality standard for Shexiang Haima Zhuifeng Gao. Centr. South Pharm. 4, 350–353.
Loh, T. L., Tewfik, A., Aylesworth, L., and Phoonsawat, R. (2016). Species in wildlife trade: socio-economic factors influence seahorse relative abundance in Thailand. Biol. Conserv. 201, 301–308. doi: 10.1016/j.biocon.2016.07.022
Lourie, S. A., Foster, S. J., Cooper, E. W., and Vincent, A. C. (2004). A guide to the identification of seahorses. Proj. Seah. Traffic North Am. 4:114.
Lu, Y., Chen, W. X., Hua, Y. Q., and Meng, D. C. (2001). Pharmacological research on effect of haima zhuangyang capsule in warming kidney and strengthening Yang. J. Nanjing. Med. Univ. 17, 99–101. doi: 10.14148/j.issn.1672-0482.2001.02.014
Luo, J. Y., Yan, D., Song, J. Y., Zhang, D., Xing, X. Y., Han, Y. M., et al. (2013). A strategy for trade monitoring and substitution of the organs of threatened animals. Sci. Rep. 3:3108. doi: 10.1038/srep03108
Macedo, M. W. F. S., Cunha, N.B. d., Carneiro, J. A., Costa, R. A. d., Alencar, S. A. d., Cardoso, M. H., et al. (2021). Marine organisms as a rich source of biologically active peptides. Front. Mar. Sci. 8:889. doi: 10.3389/fmars.2021.667764
Mandl, L. A. (2019). Osteoarthritis year in review 2018: clinical. Osteoarth. Cartil. 27, 359–364. doi: 10.1016/j.joca.2018.11.001
Mei, X. T., Xu, D. H., Lin, Z. L., Xu, S. B., and Li, B. J. (2005). Strengthening and restoring effects of Hippocampus Capsule on kidney-deficient rats. Chin. Tradit. Herbal Drugs 3, 409–410.
Mitsuhashi, S. (2014). Current topics in the biotechnological production of essential amino acids, functional amino acids, and dipeptides. Curr. Opin. Biotechnol. 26(Suppl. 6), 38–44. doi: 10.1016/j.copbio.2013.08.020
Murray, M., Dordevic, A. L., Ryan, L., and Bonham, M. P. (2018). An emerging trend in functional foods for the prevention of cardiovascular disease and diabetes: marine algal polyphenols. Crit. Rev. Food Sci. Nutr. 58, 1342–1358. doi: 10.1080/10408398.2016.1259209
NCBI (2020). Hippocampus [Online]. Available online at: https://www.ncbi.nlm.nih.gov/Taxonomy/taxonomyhome.html/ (accessed November 3, 2020).
Newman, D. J., and Cragg, G. M. (2016). Drugs and drug candidates from marine sources: an assessment of the current “state of play”. Planta Med. 82, 775–789. doi: 10.1055/s-0042-101353
Nicolás, V.-G., Arjona, O., Morales-Bojorquez, E., Mascaro, M., Simoes, N., and Palacios, E. (2014). Assessment of lipid classes and fatty acid levels in wild newborn seahorses (Hippocampus erectus) (Perry 1810): implications for survival and growth in aquarium culture. Mar. Behav. Physiol. 47, 401–413. doi: 10.1080/10236244.2014.959368
Otero-Ferrer, F., Molina, L., Socorro, J., Herrera, R., Fernández-Palacios, H., and Izquierdo, M. S. (2010). Live prey first feeding regimes for short-snouted seahorse Hippocampus hippocampus (Linnaeus, 1758) juveniles. Aquac. Res. 41, e8–e19. doi: 10.1111/j.1365-2109.2010.02505.x
Pangestuti, R., and Kim, S.-K. (2015). Peptide-derived from seahorse exerts a protective effect against cholinergic neuronal death in in vitro model of Alzheimer’s Disease. Proc. Chem. 14, 343–352. doi: 10.1016/j.proche.2015.03.047
Pangestuti, R., Ryu, B., Himaya, S., and Kim, S. K. (2013). Optimization of hydrolysis conditions, isolation, and identification of neuroprotective peptides derived from seahorse Hippocampus trimaculatus. Amino Acids 45, 369–381. doi: 10.1007/s00726-013-1510-4
Peng, W. D., and Chen, Q. L. (2005). Anti-fatigue effect of enzymatic extract of hippocampus. Chin. J. Food Hygiene 17, 404–407. doi: 10.13590/j.cjfh.2005.05.006
Qi, D., Qiao, Y., Zhang, X., Yu, H., Cheng, B., and Qiao, H. (2012). Aβ damages learning and memory in Alzheimer’s disease rats with kidney-yang deficiency. Evid. Based Compl. Alt. Med. 2012:132829. doi: 10.1155/2012/132829
Qian, Z. J., Kang, K. H., and Kim, S. K. (2012). Isolation and antioxidant activity evaluation of two new phthalate derivatives from seahorse, Hippocampus kuda Bleeler. Biotechnol. Bioprocess Eng. 17, 1031–1040. doi: 10.1007/s12257-012-0115-1
Qian, Z. J., Ryu, B., Kim, M. M., and Kim, S. K. (2008). Free radical and reactive oxygen species scavenging activities of the extracts from seahorse, Hippocampus kuda bleeler. Biotechnol. Bioprocess Eng 13, 705–715. doi: 10.1007/s12257-008-0093-5
Reheman, A., Gao, Z. Y., Tursun, X., Pu, X. P., Wu, T., He, F., et al. (2019). Optimization of extraction technology of majun mupakhi ela and its effect on hydrocortisone-induced kidney yang deficiency in mice. Sci. Rep. 9:4628. doi: 10.1038/s41598-019-41006-6
Rosa, I. M., Alves, R. R., Bonifácio, K. M., Mourão, J. S., Osório, F. M., Oliveira, T. P., et al. (2005). Fishers’ knowledge and seahorse conservation in Brazil. J. Ethnobiol. Ethnomed. 1:12. doi: 10.1186/1746-4269-1-12
Ryu, B. (2010). Purification of a peptide from seahorse, that inhibits TPA-induced MMP, iNOS and COX-2 expression through MAPK and NF-κB activation, and induces human osteoblastic and chondrocytic differentiation. Chem. Biol. Interact. 184, 413–422. doi: 10.1016/j.cbi.2009.12.003
Ryu, B., Qian, Z.-J., and Kim, S.-K. (2010). SHP-1, a novel peptide isolated from seahorse inhibits collagen release through the suppression of collagenases 1 and 3, nitric oxide products regulated by NF-κB/p38 kinase. Peptides 31, 79–87. doi: 10.1016/j.peptides.2009.10.019
Sable, R., Parajuli, P., and Jois, S. (2017). Peptides, peptidomimetics, and polypeptides from marine sources: a wealth of natural sources for pharmaceutical applications. Mar. Drugs 15:124. doi: 10.3390/md15040124
Sarlus, H., and Heneka, M. T. (2017). Microglia in Alzheimer’s disease. J. Clin. Invest. 127, 3240–3249. doi: 10.1172/JCI90606
Segade, A., Robaina, L., Novelli, B., Otero-Ferrer, F., and Dominguez, L. M. (2016). Effect of the diet on lipid composition and liver histology of short snout seahorse Hippocampus hippocampus. Aquacult. Nutr. 22, 1312–1319. doi: 10.1111/anu.12341
She, M., He, G. X., Chen, H., and Jin, Z. J. (1995). An experimental study of 5 kinds of halibios on antiageing action. Chin. J. Mar. Drugs 2, 30–34. doi: 10.13400/j.cnki.cjmd.1995.02.008
Shen, Q., Dai, Z., Huang, Y. W., and Cheung, H. Y. (2016). Lipidomic profiling of dried seahorses by hydrophilic interaction chromatography coupled to mass spectrometry. Food Chem. 205, 89–96. doi: 10.1016/j.foodchem.2016.02.151
Sheng, L., Luo, H. Y., Zhang, J. Q., and Liu, M. S. (2011). Study on processing method of Hippocampus preparation. Chin. Med. J. Res. Prac. 25, 55–57. doi: 10.13728/j.1673-6427.2011.02.023
Sherman, A. R. (1992). Zinc, copper, and iron nutriture and immunity. J. Nutr. 122(Suppl. 3), 604–609. doi: 10.1093/jn/122.suppl_3.604
Shi, J., Su, R. Q., Zhang, W. T., and Chen, J. (2020). Purification and the secondary structure of a novel angiotensin I-converting enzyme (ACE) inhibitory peptide from the alcalase hydrolysate of seahorse protein. J. Food Sci. Technol. 57, 3927–3934. doi: 10.1007/s13197-020-04427-0
Si, X. D., Ge, X. M., Xu, Y. J., and Wang, R. P. (2018). Chemical composition analysis of Hippocampus kuda bleeker based on odor objectification. J.Nuc. Agricult. Sci. 32, 941–951.
Song, L. R. (1999). Chinese Materia Medica (Selected Edition). Shanghai: Shanghai Scientific & Technical Publishers.
Su, Y. T., and Xu, Y. J. (2015a). Study on the extraction and purification of glycoprotein from the yellow seahorse, Hippocampus kuda Bleeker. Food Sci. Nutr. 3, 302–312. doi: 10.1002/fsn3.219
Su, Y. T., and Xu, Y. J. (2015b). Study on differences of fatty acids from the farmed and wild seahorses, Hippocampus kuda bleeker. Chin. J. Mar. Drugs 34, 7–12. doi: 10.13400/j.cnki.cjmd.2015.04.002
Sun, D., Wu, S., Jing, C., Zhang, N., Liang, D., and Xu, A. (2012). Identification, synthesis and characterization of a novel antimicrobial peptide HKPLP derived from Hippocampus kuda bleeker. J. Antibiot. (Tokyo) 65, 117–121. doi: 10.1038/ja.2011.120
Sun, H. H. (2015). The Effect and Mechanism of Sterol in Hippocampus kuda on Mice Reproductive System. Master’s thesis. Hangzhou: Zhejiang Gongshang University.
Sun, J. H., Xia, S. D., Xie, S. D., Yang, Y. Q., Cui, P., Shao, P., et al. (2020). Biochemical composition of wild and cultured seahorses (Hippocampus kuda bleeker). Aquac. Res. 51, 1542–1550. doi: 10.1111/are.14502
TCMID (2020). Hippocampus [Online]. Available online at: http://47.100.169.139/tcmid/herb/7468 (accessed December 18, 2020).
Tian, J., Huang, Y., Wu, T., Huang, H. D., Ko, K. M., Zhu, B. T., et al. (2021). The use of chinese Yang/Qi-invigorating tonic botanical drugs/herbal formulations in ameliorating chronic kidney disease by enhancing mitochondrial function. Front. Pharmacol. 12:622498. doi: 10.3389/fphar.2021.622498
Vincent, A. C., Foster, S. J., and Koldewey, H. J. (2011). Conservation and management of seahorses and other syngnathidae. J. Fish. Biol. 78, 1681–1724. doi: 10.1111/j.1095-8649.2011.03003.x
Wang, G. F., Dong, J. C., and Duan, X. H. (2012). Concept of “kidney” in TCM and mechanism, method, prescription, drug & application of kidney-invigoration. Chin. J. Tradit. Chin. Med. Pharm. 27, 3112–3115.
Wang, Q., Zhang, Z. H., Zang, X. X., and Xu, G. J. (1998). Chemical studies on Hippocampus histrix kaup. J. Chin. Pharm. Univ. 29, 3–5.
Wang, X. Y. (2015). Research on Identification and Anti-Osteoporosis Activity of Traditional Chinese Medicine Hippocampus. Master’s thesis. Fuzhou: Fujian University of Traditional Chinese Medicine.
Watanabe, Y., and Tatsuno, I. (2017). Omega-3 polyunsaturated fatty acids for cardiovascular diseases: present, past and future. Exp. Rev. Clin. Pharmacol. 10, 865–873. doi: 10.1080/17512433.2017.1333902
Wei, N., Xu, W. W., Wei, Q., Gao, B. M., and Wang, Y. (2015). Assay of nucleosides and nucleotides in Hippocampus by HPLC. Guang. Chem. Industry 43, 128–130.
Wu, J. N., Liu, Z. Y., Su, J., Lu, H. X., Liao, D. Y., and Song, Q. L. (2017b). Chemical constituents of the seahorse Hippocampus trimaculatus from East China Sea. Chem. Nat. Compd. 53, 982–983. doi: 10.1007/s10600-017-2178-x
Wu, J., Chen, X., Pan, N., Chen, B., Zhang, J., and Liu, Z. (2020). 3beta-Hydroxycholest-5-en-7-one from seahorse alleviates lipopolysaccharide-induced inflammatory responses by downregulating miR-98-5p. Life Sci. 258:118176. doi: 10.1016/j.lfs.2020.118176
Wu, J. N., Liu, Z., Su, J., Pan, N., and Song, Q. (2017a). Anti-inflammatory activity of 3beta-hydroxycholest-5-en-7-one isolated from Hippocampus trimaculatus leach via inhibiting iNOS, TNF-alpha, and 1L-1beta of LPS induced RAW 264.7 macrophage cells. Food Funct. 8, 788–795. doi: 10.1039/c6fo01154c
Wu, Q., Sun, J., Chen, J., Zhang, H., Guo, Y. W., and Wang, H. (2018). Terpenoids from marine soft coral of the genus lemnalia: chemistry and biological activities. Mar. Drugs 16:320. doi: 10.3390/md16090320
Xu, D. H., and Xu, S. B. (1997). The extract from hippocampus trimaculatus(eht): its effect of antithrombosis in rats and ingredients analysis. Chin. J. Mar. Drugs 1, 11–13. doi: 10.13400/j.cnki.cjmd.1997.01.004
Xu, D. H., Wang, L. H., Mei, X. T., Li, B. J., Lv, J. L., and Xu, S. B. (2014). Protective effects of seahorse extracts in a rat castration and testosterone-induced benign prostatic hyperplasia model and mouse oligospermatism model. Environ. Toxicol. Pharmacol. 37, 679–688. doi: 10.1016/j.etap.2014.02.001
Xu, K. H. (2015). Study on ACE-Inhibitory and Antioxidant Activities of Three-Spot Hippocampus Hydrolysis Peptides. Master’s thesis. Haikou: Hainan University.
Xu, S. B., Xu, D. H., Lv, J. Y., Mei, X. T., Wu, Z. F., and Li, B. J. (2002). Prospect of research and development for Chinese medicinal material Hippocampus in China. Chin. Tradit. Herbal Drugs 33, 82–84.
Xu, Y. M., Chen, J. W., and Guo, R. (1994). Studies on the phospholipids and fatty acids in traditional chinese medicinal materials hippocampus and syngnathus. Chin. J. Mar. Drugs 4, 14–18. doi: 10.13400/j.cnki.cjmd.1994.01.003
Xu, Y., Ryu, S., Lee, Y. K., and Lee, H. J. (2020). Brassicasterol from edible aquacultural hippocampus abdominalis exerts an anti-cancer effect by dual-targeting AKT and AR signaling in prostate cancer. Biomedicines 8:370. doi: 10.3390/biomedicines8090370
Yan, L. (2019). The Relationship Between Inflammation and Autophagy and Autophagy Related mTOR Signaling Pathway in Schizophrenia and the Anti-Neuroinflammatory Effect of Hippocampus erectus Ethanol Extracts. Master’s thesis. Zhanjiang: Guangdong Ocean University.
Yan, L., Yang, Z. Y., Huang, W. Z., Zhang, Y. P., Yang, W. C., Liu, Y. Y., et al. (2019). Anti-inflammatory and Nitric Oxide(NO) Release Effects of Hippocampus erectus Ethanol Extracts on Lipopolysaccharide-induced BV2 Microglia. J. Guang. Ocean Univ. 39, 90–96. doi: 10.3969/j.issn.1673-9159.2019.01.014
Yan, Z. Z. (2019). Preliminary Studies on Chemical Ecology of Biochemical Composition in Two Seahorses, Hippocampus erectus and H. trimaculatus. Master’s thesis. Shanghai: Shanghai Ocean University.
Yang, Y., Wang, Z., Gu, Y. L., Xu, Q. P., and Kong, Y. (2014). Study on chemical constituents of Hippocampus erinaceus. J. China Pharm. 25, 1780–1782. doi: 10.6039/j.issn.1001-0408.2014.19.17
Yi, M. H., Li, G. Q., Xiao, H., Lin, Z. S., and Zeng, D. Z. (2006). Study on the antioxidative activities of extracts of Hippocampus trimaculatus leach and syngnathus on fat and oil. China Trop. Med. 6, 1784–1785.
Yu, M. M. (2018). Study on Preparation and Anti-Tumor Activity of Sphingoid Bases from Hippocampus Trimaculatus Leach. Master’s thesis. Haikou: Hainan University.
Yuan, X. H., Chen, C. B., Chen, Z., and Yi, M. H. (2011). Study on optimization of enzymatic and the antioxidant activity to DPPH of liquid protein of Three-spot hippocmpus. Sci. Technol. Food Industry 32, 291–293. doi: 10.13386/j.jssn1002-0306.2011.09.032
Zhang, D. B. (2021). The meaning and significance of the strict regularity of the concept of “Yin and Yang”. Tradit Chin. Med. J. 20, 1–3. doi: 10.14046/j.cnki.zyytb2002.2021.01.002
Zhang, Q., and Wang, Y. L. (1996). Extraction and analysis of fat of S. acus linnaeus and H. japonicus kaup. Chin. J. Anal. Chem. 24, 139–143.
Zhang, Z. H., Xu, G. J., Xu, L. S., and Wang, Q. (1995). Hormonic effects of the ethanol extracts of syngnathus. J. Chin. Med. Mater. 18, 197–199. doi: 10.13863/j.issn1001-4454.1995.04.018
Zhang, Z. H., Xu, G. J., Xu, L. S., and Wang, Q. (1997). Physical and chemical analysis of medicinal animals of syngnathidae. J. Chin. Med. Mater. 20, 140–144. doi: 10.13863/j.issn1001-4454.1997.03.012
Zhang, Z. H., Xu, G. J., Xu, L. S., and Wang, Q. (1998). Analysis of steroids in crude drugs by capillary gas chromatography. J. Chin. Med. Mater. 21, 81–83. doi: 10.13863/j.issn1001-4454.1998.02.013
Zhang, Z., Gao, T. H., Fu, C. M., Zhang, J. M., Shi, J. F., He, Y., et al. (2017). Analysis on dosage form theory and current application situation of traditional Chinese medicine pill. Chin. J. Chin. Mater. Med. 42, 2408–2412. doi: 10.19540/j.cnki.cjcmm.20170416.001
Zhao, X. F., Xiang, G. X., Shi, C. W., Wang, M. Y., Fu, X. J., and Teng, J. L. (2021). Exploration of marine traditional chinese medicine experience in the use of hippocampus through the ages based on knowledge discovery and associative network construction of formulary composition information. Modern. Trad. Chin. Med. Mat. Med. World Sci. Technol. 23, 335–343.
Zhao, X. Z. (2018). Study on the Otherness of Chemical Constituents in Hippocampus of Different Commodity Medicinal Materials by Metabolomics Approach. Master’s thesis. Taiyuan: Shanxi University.
Zheng, L., Chen, J., Zhao, H., Shi, Q., Yang, B., Cheng, H., et al. (2012). Rapid finding and quantification of the major antioxidant in water extracts of three marine drug organisms from China by online HPLC-DAD/MS-DPPH. Nat. Prod. Res. 26, 873–877. doi: 10.1080/14786419.2011.565285
Zhi, D. G., Pang, J. P., Jin, Z. X., Chen, F. R., Wang, Y. J., Fan, D., et al. (2019a). Effect of haima bushen pills on nourishing yin and reinforcing kidney. China Tradit. Herb Drugs 50, 2632–2638. doi: 10.7501/j.issn.0253-2670.2019.11.019
Zhi, D. G., Pang, J. P., Jin, Z. X., Chen, F. R., Wang, Y. J., Fan, D., et al. (2019b). Improvement of haima bushen pills on learing and memory impairment in mice. Drugs Clin. 34, 1290–1293. doi: 10.7501/j.issn.1674-5515.2019.05.005
Zhu, A. M. (2004). Study of toxicity of ethanol extracts from Hippocampus. China Pharm. 10, 822–824.
Keywords: Hippocampus, traditional marine Chinese medicine, resources, active ingredients, pharmacological activities
Citation: Cui X, Zhao X, Li J, Li Z, Ren X, Zhao Y, Fu X and Li X (2021) Advances in the Global Distribution, Chemical Constituents, and Pharmacology of Hippocampus, a Traditional Marine Chinese Medicine. Front. Mar. Sci. 8:774927. doi: 10.3389/fmars.2021.774927
Received: 13 September 2021; Accepted: 30 October 2021;
Published: 06 December 2021.
Edited by:
Bin Wu, Zhejiang University, ChinaReviewed by:
Yueying Li, Texas A&M University, United StatesCaisheng Wu, Xiamen University, China
Qihao Wu, Yale University, United States
Copyright © 2021 Cui, Zhao, Li, Li, Ren, Zhao, Fu and Li. This is an open-access article distributed under the terms of the Creative Commons Attribution License (CC BY). The use, distribution or reproduction in other forums is permitted, provided the original author(s) and the copyright owner(s) are credited and that the original publication in this journal is cited, in accordance with accepted academic practice. No use, distribution or reproduction is permitted which does not comply with these terms.
*Correspondence: Xianjun Fu, xianxiu@hotmail.com; Xiuxue Li, lixiuxuelixiuxue@126.com